Weightlessness damaged the ultrastructure of knee cartilage and quadriceps muscle, aggravated the degeneration of cartilage
Highlight box
Key findings
• Through tail suspension simulation of weightlessness, it was found that weightlessness caused damage to cartilage endoplasmic reticulum and mitochondria, loss of cartilage collagen fibers and water, increase of joint inflammatory factors, and acceleration of cartilage degeneration.
• The weightless environment also causes damage to muscle mitochondria, and muscle mitochondria lose their activity.
• The elasticity and hardness of cartilage are decreased, and the micro-surface becomes uneven.
What is known and what is new?
• In weightlessness, cartilage degeneration and muscle atrophy accelerate.
• Weightlessness leads to the damage of cartilage mitochondria, the decrease of cartilage elastic modulus and hardness, the damage of cartilage surface and the aggravation of degeneration. The activity of muscle mitochondria is decreased and damaged.
What is the implication, and what should change now?
• In space or bedridden environment, long-term weightlessness may lead to cartilage degeneration, damage to cartilage mitochondria, aggravated degeneration and damage to muscle mitochondria activity. It is recommended that prolonged weightlessness should be avoided, and if it is unavoidable, appropriate treatment measures (such as appropriate weight-bearing and vibration therapy, etc.) should be taken to improve the damage caused by weightlessness.
Introduction
Musculoskeletal complications from weightlessness pose a major challenge to clinicians and National Aeronautics and Space (NASA), as long-term weightlessness induces bone and muscle degradation that can ultimately lead to musculoskeletal system failure (1-3). In addition to the muscles and bones, the knee’s soft tissue structures, which include articular cartilage, meniscus, tendons, and ligaments, are vulnerable to changes in loading conditions (4,5). A study by Kwok et al. (6) demonstrated that both tail suspension and total body irradiation induce acute degeneration of the knee joint, with partial recovery of cartilage degeneration observed upon resumption of normal weight bearing. Tsai et al. showed that limb immobilization caused cartilage degeneration after knee meniscus surgery in rats (7). Muscle tissues also exhibit high sensitivity to loading conditions, with studies illustrating varying degrees of muscle atrophy during prolonged weightlessness, which significantly impairs functionality (8). In clinical practice, many cases require patients to be in a bedridden state, which will cause patients to lose the stimulation of the knee joint load, thus aggravating the degeneration of articular cartilage (9,10). Although the exact extent of damage to the knee’s soft tissues resulting from prolonged unloading remains uncertain, substantial evidence indicates that reduced mechanical loads lead to articular cartilage degradation and muscle atrophy (11-13). The present study employed a rat model simulating weightlessness environment using the hindlimb unloading (HLU) technique to investigate the impact of a weightlessness on the ultrastructure of the quadriceps femoris muscle and knee joint cartilage in rats. Research hypothesis: (I) suspension of the tail will cause the destruction of the cartilage structure of the knee joint and aggravate the degeneration of cartilage; (II) tail suspension will cause damage to the mitochondrial structure of the quadriceps muscle and loss of activity. We present this article in accordance with the ARRIVE reporting checklist (available at https://aoj.amegroups.com/article/view/10.21037/aoj-24-6/rc).
Methods
Study design
A total of 30 male Sprague-Dawley rats, aged 16 weeks, were procured from Beijing Vitahe Experimental Animal Technology Co., LTD., China. Each rat was individually housed in separate cages within a temperature-controlled environment and maintained on a 12-hour light-dark schedule, with ad libitum access to food and water. Following a 5-day acclimation period, the rats were grouped based on their weight, with 20 rats randomly selected from the initial 30. These 20 rats were further divided into two groups, with one group subjected to tail suspension for 14 days and the other for 28 days, in order to induce hind limb unloading. The remaining ten rats were kept under normal loading conditions within their cages. Therefore, three groups (10 rats/groups) were established: the ground group, the 14-day HLU group, and the 28-day HLU group (Figure 1A). Male Sprague-Dawley rats were chosen since they are frequently used on shuttle missions where space is limited. Experiments were performed under a project license (No. A2023120) granted by the Animal Management and Use Committee of Peking University Health Science Center, in compliance with Peking University Health Science Center institutional guidelines for the care and use of animals.
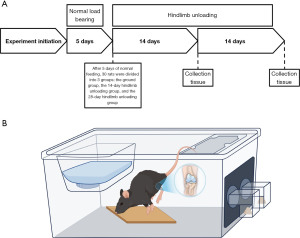
HLU procedure
On the fifth day of the experiment, 14- and 28-day HLU rats were lightly anesthetized with ketamine/xylazine while in their cages, and the Morey-Holton method, as described in previous studies (14,15), was employed to simulate weightlessness. To achieve this, medical tape was carefully applied around the tail of each rat, creating a slip-knot. The tail was then lifted around the top beam of the suspension device, resulting in a position where the rat’s head was lowered, the forelimb supported a portion of the weight, the hind limb was elevated off the ground, and the long axis of the body formed a 30° angle with the horizontal plane. Throughout the suspension, the rats were allowed to freely consume water and move their heads (Figure 1B).
Tissue harvest
On either the 14th or 28th day of the experiment, the rats were anesthetized with 3% sevoflurane inhalation anesthesia. The left knee joint was subjected to magnetic resonance imaging (MRI) to assess T2 mapping. The skin of the right hind limb was incised, allowing for the isolation of the quadriceps muscle and exposure of the knee joint. Subsequently, the patellar ligaments on both sides were dissected using blades, fully exposing the tibial plateau and femoral condyles. Joint fluid was then extracted for analysis. Following the extraction, a portion of the knee joint was collected, and small pieces of the cartilage from the femoral condyle were rapidly excised using a scalpel, with dimensions of approximately 1 mm3 each. Cross-sectional samples of the quadriceps femoris muscle, measuring approximately 1 cm × 1 mm × 1 mm, were also obtained for transmission electron microscope analysis. After the aforementioned procedures, the remaining rat knee joints were fixed in a 4% paraformaldehyde fixative. After 3 days of fixation, both hindlimbs were transferred to 70% ethanol and subsequently flash-frozen in liquid nitrogen. The frozen samples were stored at −80 ℃ for subsequent histological analysis and biomechanical assessment.
Primary outcome
Transmission electron microscope
Small pieces of cartilage were removed from the medial femoral region of the rat knee joint and small piece of quadriceps femoris cross-sectional muscle above the knee joint was taken. The cartilage and muscle tissues were cleansed with phosphate-buffered saline (PBS), fixed with 1% osmium acid, and further cleaned with PBS. Afterwards, the samples were dehydrated, infiltrated with resin, embedded, cured, and sectioned to a thickness of 70 nm. Eight to 10 slices were randomly selected from each group with three to six fields of view per slice for analysis. To visualize the ultrastructures, lead-uranium double staining was performed, and transmission electron microscopy was utilized to observe the ultrastructures and capture photographs.
Secondary outcomes
T2 mapping
T2 mapping is a multi-echo magnetic resonance sequence that can assess the concentration, direction and integrity of collagen and water content in cartilage matrix. It is a sensitive technique for early detection of cartilage degeneration (16,17). The experimental animals were euthanized according to the predetermined time frame outlined in the experimental plan. Subsequently, MRI scans of the joints were immediately conducted using a Discovery MR750 3.0T MR scanner (Discovery® MR750 MR System, GE Healthcare, Japan) equipped with an 8-channel knee coil (n=10 per group). See Appendix 1 for details. The average T2 relaxation times were calculated using the Bruker ParaVision 5.0 system, and the data were analyzed by a senior musculoskeletal radiologist.
Mitochondrial extraction and analysis
For mitochondrial extraction, the quadriceps muscle tissue from 30 rats was subjected to the use of a mitochondrial extraction kit (SM0020, Beijing Sora Biotechnology Co., LTD., China). Following mitochondrial extraction, the rat mitochondrial respiratory chain complex II [enzyme-linked immunosorbent assay (ELISA)] kit was employed for analysis, with specific procedures executed according to the instructions specified by the kit. See Appendix 1 for details.
Articular fluid extraction and inflammatory factor assay
After performing anesthesia on the 30 rats, the knee fluid was extracted by injecting 1 mL of physiological saline into the lateral space of the knee. Subsequently, the knee joint was stretched and flexed 10 times to facilitate the aspiration of joint fluid using a 2 mL syringe. The collected fluid was then centrifuged at 4,000 rpm and 49 ℃ for 20 minutes, and the supernatant was carefully obtained. The collected samples were stored at −80 ℃ for further analysis. See Appendix 1 for details.
Following the extraction of knee fluid, the levels of inflammatory cytokines were determined using ELISA kits for interleukin-1beta (IL-1β) (Cloud-Clone Corp; article No. SEA563Ra; batch No. L140116385) and tumour necrosis factor alpha (TNF-α) Rat ELISA Kit (Life Technologies, Lot: 1290859B; batch No. KRC3011). The results were processed using ELISA data processing software.
Histological staining and Immunohistochemistry assessment
Following the joint fluid test, the knee joint was fixed and subsequently embedded in paraffin. The knee joints from each group were then sliced in the sagittal plane (5 µm thick). These sections were stained with alcian blue to qualitatively evaluate the overall morphology, as well as safranin O to assess the presence of Proteoglycan content and arthritis. The subsequent steps involved in the process included knee fixation, decalcification, paraffin embedding, tissue sectioning For immunohistochemistry, the tissue sections were subjected to a 5-minute treatment with a 3% hydrogen peroxide solution, followed by a 30-minute antigen retrieval process using Pepsin at a temperature of 37 ℃. Subsequently, the sections were incubated overnight at 4 ℃ with a diluted solution of Mouse collagen type 11 antibody (1:50) in PBS. To facilitate color development, the sections underwent diaminobenzidine (DAB) staining, after which a distilled water wash was performed to eliminate any residual color. The nuclei were restained with Hematoxylin, resulting in a blue color separation. Gradual dehydration was achieved through the use of alcohol gradients, followed by xylene-based transparency. Finally, the sections were sealed with neutral gum. Five slides were randomly selected from each group, and Image Pro Plus 6.0 software was used for quantitative analysis of immunohistochemical staining images. All image assessments were performed by three blinded observers, and quantitative data were presented as the mean of three assessments.
Nanoindentation evaluation of cartilage
The biomechanical features of cartilage, including microscopic geomorphology, elastic modulus, and hardness, were assessed using nanoindentation. Cartilage samples (n=6 per group) were harvested from the condyle of femur of animals in each group. Circumfluent PBS solution was used to maintain hydration during the tests. A TriboIndenter (Hysitron Inc., Minneapolis, MN, USA) with a conospherical diamond probe tip with 400-mm radial curvature was applied to evaluate the samples. Each indent site was tested using a trapezoidal load function that included loading (10 seconds), hold (2 seconds), and unloading (10 seconds). The maximum depth of indentation was 500 nm. The parameters of reduced modulus and hardness in different groups were assessed and calculated. Meanwhile, the nano-scale microstructure of the surface of the indentation zone was observed using the micro-scanning equipment that comes with the equipment itself to observe the micro-surface morphology.
Statistical analysis
All data were represented as the mean ± standard deviation. Data were analyzed using SPSS 26.0 (SPSS for Windows, version 26, SPSS Inc., Chicago, IL, USA). One-way analysis of variance (three groups or more) or two-tailed t-test (unpaired, two groups) was used for statistical analysis followed by post hoc analysis using Tukey’s HSD test. Statistical significance was defined as P<0.05.
Results
Ultrastructure of cartilage and quadriceps femoris muscle
The ultrastructural analysis of cartilage revealed that knee chondrocytes in the ground group exhibited a normal morphology in terms of mitochondria and rough endoplasmic reticulum. Additionally, the mitochondrial cristae were observed to be intact. However, after a period of 2 weeks in a weightless environment, the knee cartilage cells showed mitochondrial swelling or complete absence, while the size of the rough endoplasmic reticulum (ER) decreased and the lumen enlarged (P<0.05). After a period of 4 weeks in a weightless environment, observations revealed a reduction or absence of mitochondrial cristae, evident swelling or disappearance of mitochondria, and a noticeable decrease in the rough surface endoplasmic reticulum (P<0.05) (Figure 2A). Compared with the control group, the muscle ultrastructure analysis showed that the mitochondrial ridge was weakened in the weightlessness group for 14 days, weightlessness for 28 days, mitochondria swelling, cavitation, mitochondria ridge weakenss (P<0.05) (Figure 2B).
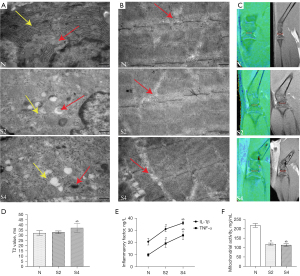
T2 mapping
No significant changes were observed in the tibiofemoral cartilage contact area of the medial knee compartment on T2 mapping and conventional sequences at 14 days. On day 28, T2 mapping observed significant changes in the tibia-femoral cartilage contact area in the medial knee joint. The T2 value of HLU rats was slightly higher than that of ground rats, indicating the loss of water and collagen fibers. Cartilage thinning in the medial region was observed with conventional sequences (Figure 2C). The quantitative results of T2 analysis showed that the T2 value of the 28-day weightlessness group was higher than that of the 14-day weightlessness group and the normal group (Figure 2D).
Joint fluid inflammatory factors and mitochondrial activity
In comparison to the ground group, the knee fluid of the HLU group exhibited a significant increase in the concentrations of IL-1β and TNF-α (P<0.05). Furthermore, the level of inflammatory factors in the 28-day HLU group was significantly higher than that in the 14-day HLU group (P<0.05) (Figure 2E). This indicates that with the extension of weightlessness, the level of inflammatory factors in the joint fluid is increased. The findings from standardized experiments on mitochondrial complex II indicated varying degrees of damage to the mitochondrial activity in the quadriceps femoris muscle of rats. The quadriceps femoris muscle activity of rats in the HLU group exhibited a significant decrease compared to the ground group. Additionally, the mitochondrial activity in the 28-day HLU group was found to be lower than that in the 14-day HLU group (Figure 2F). This decrease in activity is indicative of impaired mitochondrial function.
Histological results
To investigate the impact of weightless conditions on cartilage, the evaluation of safranin O and alcian blue staining was conducted on the knee joint of rats, which was designed to assess the overall morphology. The morphology and structure of articular cartilage in normal control group were basically the same. In contrast, there was a slight cartilage defect in the knee after 14 days of tail suspension, there was a slight cartilage defect in the knee joint. Subsequently, at the 28-day mark, cartilage defects become apparent (Figure 3A,3B).
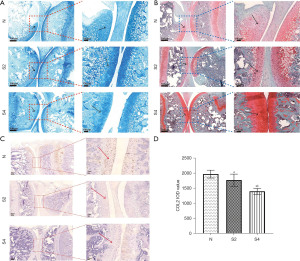
Immunohistochemical staining was used to observe the effect of weightlessness on COL2 expression in knee cartilage, and also showed obvious cartilage injury, erosion, loss of proteoglycan and impaired cell function (Figure 3C).
The staining and quantification outcomes revealed a significant reduction in COL2 expression and glycosaminoglycan (GAG) content in the HLU group compared with the ground group, and the decline degree in the group with 28-day HLU group was higher than that in the group with 14-day HLU group (Figure 3D).
Nanoindentation evaluation of cartilage
By nanoindentation biomechanical analysis, we observed a decrease in both reduced modulus and hardness in the HLU group compared with the ground group (Table 1, Figure 4A,4B). The reduction of modulus and hardness in the 28-day HLU group was higher than that in the 14-day HLU group. Using micro-scanning equipment, we observed that the cartilage surface of the HLU group was rougher and more heterogeneous than that of normal joints. In contrast, the cartilage surface of the ground group was smooth and uniform (Figure 4C).
Table 1
Category | N | S2 | S4 | P value |
---|---|---|---|---|
Reduced modulus (MPa) | 21.67±1.77 | 17.01±0.76 | 13.25±1.21 | <0.05 |
Hardness (KPa) | 4,041±206.40 | 3,725±53.56 | 3,589.67±18.92 | <0.05 |
Data are shown as the mean ± SD; SD, standard deviation; N, ground group; S2, 14-day hindlimb unloading group; S4, 28-day hindlimb unloading group.
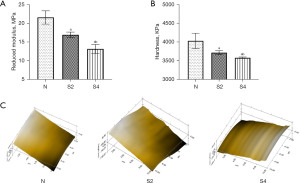
Discussion
The main findings of this study are that tail suspension simulated weightlessness seriously damaged the soft tissue structure around the knee joint, the rough endoplasmic reticulum and mitochondria of cartilage were seriously damaged, the surface fracture was uneven, the positive expression of collagen type II in cartilage was decreased, the elastic modulus and hardness of cartilage were reduced, muscle mitochondria were seriously damaged, there were obvious vacuoles, and a large number of muscle mitochondria were inactivated.
The study of load reduction on the musculoskeletal system has been a prominent topic in the aerospace and clinical fields (18,19). The musculoskeletal system is highly susceptible to changes in load-bearing, and a decrease in load can lead to detrimental effects on the system, such as joint degeneration (20,21), meniscus degeneration (22), and osteoporosis (23). Numerous studies have demonstrated the impact of reduced knee load on articular cartilage, resulting in a decrease in GAG content, as well as surface defects in the cartilage (6,20,21). Additionally, previous research has demonstrated that weightlessness induces an arthritic response in the articular cartilage of the medial tibial plateau and the meniscus in the knee due to reduced weight bearing (24). Through the examination of the ultrastructure of cartilage and quadriceps femoris, this study provides further confirmation that weightlessness exacerbates the deterioration of cartilage and muscle structure, consequently impacting the functionality of the skeletal system.
Weightlessness is relatively common in many scenarios, such as orthopedic postoperative braking and in space environments. Reduced weight bearing leads to a range of musculoskeletal complications that are a hot topic of research that needs to be urgently tackled by mankind. Braking for a long time of the human body after orthopedic surgery can lead to muscle atrophy and cartilage degeneration. Kakehi et al. recruited healthy subjects and added blood flow restriction during 14 days of braking, and the results showed that blood flow restriction reduced muscle atrophy and increased MuRF1 expression level (25). Takahashi et al. also used a caudal suspension model to reduce weight bearing and their findings suggest that physiological reloading restores the disuse atrophy of the articular cartilage and bone (26). However, the current literature is still limited due to the limitations of the research setting and equipment.
In clinical practice, many patients need to undergo prolonged bed rest to promote the recovery of damaged tissues. However, this study found that long-term weight-bearing reduction led to pathological changes in the ultrastructure of cartilage and muscle, and aggravated cartilage degradation. Therefore, appropriate rehabilitation nursing interventions such as (appropriate weight bearing, passive mobilization, vibration therapy and blood flow restriction, etc.) should be taken into account for cartilage and muscle performance during prolonged bed rest. Notably, exercise has been identified as a viable approach to enhance articular cartilage, particularly in the hip and knee joints (26). Additional therapeutic measures may involve the use of pharmacological interventions, such as recombinant human fibroblast growth factor 18 (FGF-18), a fibroblast growth factor that has demonstrated the ability to promote cartilage relaxation growth based on preliminary trial findings (27). Increased vibratory interventions during immobilization may also increase cartilage thickness (28). In the upcoming era, designing new therapeutic and preventive measures for musculoskeletal system complications caused by weightlessness is necessary to enable individuals with musculoskeletal system complications due to weightlessness to effectively manage the effects of weightlessness on the human musculoskeletal system through proper exercise, nutrition, and rehabilitation. The study has some limitations. The tail suspension method may simulate a greater weight loss environment than a person in a weightless environment. Second, the sample size of this study was limited, focusing on the effects of weightlessness on the ultrastructure of cartilage and muscle, and no corresponding therapeutic intervention was implemented. Therefore, future research work is expected to explore the effects of a new treatment regimen on muscle and cartilage recovery in weightlessness. Despite its limitations, this study provides valuable information for the progression of weight-lessening induced arthritis. It presents new findings about the microscopic characteristics of weightless cartilage, in particular highlighting severe damage to the rough endoplasmic reticulum and mitochondria. In addition, we also studied the effect of weight loss on the mitochondria of the quadriceps muscle of rats, and found that the number of muscle mitochondria decreased and accompanied by obvious vacuolation under weightlessness.
Conclusions
The weightless environment resulted in the damage of endoplasmic reticulum and mitochondria of cartilage, mitochondrial damage of quadriceps muscle, inactivation of muscle mitochondria, increased intra-articular inflammation, decreased elastic modulus and hardness, and damaged cartilage surface, which aggravated cartilage degeneration.
Acknowledgments
Funding: This work was supported by
Footnote
Reporting Checklist: The authors have completed the ARRIVE reporting checklist. Available at https://aoj.amegroups.com/article/view/10.21037/aoj-24-6/rc
Data Sharing Statement: Available at https://aoj.amegroups.com/article/view/10.21037/aoj-24-6/dss
Peer Review File: Available at https://aoj.amegroups.com/article/view/10.21037/aoj-24-6/prf
Conflicts of Interest: All authors have completed the ICMJE uniform disclosure form (available at https://aoj.amegroups.com/article/view/10.21037/aoj-24-6/coif). The authors have no conflicts of interest to declare.
Ethical Statement: The authors are accountable for all aspects of the work in ensuring that questions related to the accuracy or integrity of any part of the work are appropriately investigated and resolved. Experiments were performed under a project license (No. A2023120) granted by the Animal Management and Use Committee of Peking University Health Science Center, in compliance with Peking University Health Science Center institutional guidelines for the care and use of animals.
Open Access Statement: This is an Open Access article distributed in accordance with the Creative Commons Attribution-NonCommercial-NoDerivs 4.0 International License (CC BY-NC-ND 4.0), which permits the non-commercial replication and distribution of the article with the strict proviso that no changes or edits are made and the original work is properly cited (including links to both the formal publication through the relevant DOI and the license). See: https://creativecommons.org/licenses/by-nc-nd/4.0/.
References
- Lang T, LeBlanc A, Evans H, et al. Cortical and trabecular bone mineral loss from the spine and hip in long-duration spaceflight. J Bone Miner Res 2004;19:1006-12. [Crossref] [PubMed]
- Harrison BC, Allen DL, Girten B, et al. Skeletal muscle adaptations to microgravity exposure in the mouse. J Appl Physiol (1985) 2003;95:2462-70. [Crossref] [PubMed]
- Hedge ET, Patterson CA, Mastrandrea CJ, et al. Implementation of exercise countermeasures during spaceflight and microgravity analogue studies: Developing countermeasure protocols for bedrest in older adults (BROA). Front Physiol. 2022;13:928313. [Crossref] [PubMed]
- Vanwanseele B, Eckstein F, Knecht H, et al. Longitudinal analysis of cartilage atrophy in the knees of patients with spinal cord injury. Arthritis Rheum 2003;48:3377-81. [Crossref] [PubMed]
- Hinterwimmer S, Krammer M, Krötz M, et al. Cartilage atrophy in the knees of patients after seven weeks of partial load bearing. Arthritis Rheum 2004;50:2516-20. [Crossref] [PubMed]
- Kwok AT, Moore JE, Rosas S, et al. Knee and Hip Joint Cartilage Damage from Combined Spaceflight Hazards of Low-Dose Radiation Less than 1 Gy and Prolonged Hindlimb Unloading. Radiat Res 2019;191:497-506. [Crossref] [PubMed]
- Tsai LC, Cooper ES, Hetzendorfer KM, et al. Effects of treadmill running and limb immobilization on knee cartilage degeneration and locomotor joint kinematics in rats following knee meniscal transection. Osteoarthritis Cartilage 2019;27:1851-9. [Crossref] [PubMed]
- Zhu M, Liu Z, Gao M, et al. The effect of Bu Zhong Yi Qi decoction on simulated weightlessness-induced muscle atrophy and its mechanisms. Mol Med Rep 2017;16:5165-74. [Crossref] [PubMed]
- Liphardt AM, Godonou ET, Dreiner M, et al. Immobilization by 21 days of bed rest results in type II collagen degradation in healthy individuals. Osteoarthritis Cartilage 2024;32:177-86. [Crossref] [PubMed]
- Reiter AJ, Schott HR, Castile RM, et al. Early Joint Use Following Elbow Dislocation Limits Range-of-Motion Loss and Tissue Pathology in Posttraumatic Joint Contracture. J Bone Joint Surg Am 2023;105:223-30. [Crossref] [PubMed]
- Souza RB, Baum T, Wu S, et al. Effects of unloading on knee articular cartilage T1rho and T2 magnetic resonance imaging relaxation times: a case series. J Orthop Sports Phys Ther 2012;42:511-20. [Crossref] [PubMed]
- Luan HQ, Sun LW, Huang YF, et al. Use of micro-computed tomography to evaluate the effects of exercise on preventing the degeneration of articular cartilage in tail-suspended rats. Life Sci Space Res (Amst) 2015;6:15-20. [Crossref] [PubMed]
- Ramkumar PN, Navarro SM, Becker J, et al. The Effects of Space Microgravity on Hip and Knee Cartilage: A New Frontier in Orthopaedics. Surg Technol Int 2019;35:421-5. [PubMed]
- Rubinstein L, Paul AM, Houseman C, et al. Placenta-Expanded Stromal Cell Therapy in a Rodent Model of Simulated Weightlessness. Cells 2021;10:940. [Crossref] [PubMed]
- Morey-Holton ER, Globus RK. Hindlimb unloading rodent model: technical aspects. J Appl Physiol (1985) 2002;92:1367-77. [Crossref] [PubMed]
- Prasad AP, Nardo L, Schooler J, et al. T1ρ and T2 relaxation times predict progression of knee osteoarthritis. Osteoarthritis Cartilage 2013;21:69-76. [Crossref] [PubMed]
- Spandonis Y, Heese FP, Hall LD. High resolution MRI relaxation measurements of water in the articular cartilage of the meniscectomized rat knee at 4.7 T. Magn Reson Imaging 2004;22:943-51. [Crossref] [PubMed]
- Stepanek J, Blue RS, Parazynski S. Space Medicine in the Era of Civilian Spaceflight. N Engl J Med 2019;380:1053-60. [Crossref] [PubMed]
- Lang T, Van Loon JJWA, Bloomfield S, et al. Towards human exploration of space: the THESEUS review series on muscle and bone research priorities. NPJ Microgravity 2017;3:8. [Crossref] [PubMed]
- Reynolds RJ, Walker PS, Buza J. Mechanisms of anterior-posterior stability of the knee joint under load-bearing. J Biomech 2017;57:39-45. [Crossref] [PubMed]
- Fitzgerald J, Endicott J, Hansen U, et al. Articular cartilage and sternal fibrocartilage respond differently to extended microgravity. NPJ Microgravity 2019;5:3. [Crossref] [PubMed]
- Ma Z, Li DX, Chee RKW, et al. Mechanical Unloading of Engineered Human Meniscus Models Under Simulated Microgravity: A Transcriptomic Study. Sci Data 2022;9:736. [Crossref] [PubMed]
- Sibonga J, Matsumoto T, Jones J, et al. Resistive exercise in astronauts on prolonged spaceflights provides partial protection against spaceflight-induced bone loss. Bone 2019;128:112037. [Crossref] [PubMed]
- Kwok AT, Mohamed NS, Plate JF, et al. Spaceflight and hind limb unloading induces an arthritic phenotype in knee articular cartilage and menisci of rodents. Sci Rep 2021;11:10469. [Crossref] [PubMed]
- Kakehi S, Tamura Y, Kubota A, et al. Effects of blood flow restriction on muscle size and gene expression in muscle during immobilization: A pilot study. Physiol Rep 2020;8:e14516. [Crossref] [PubMed]
- Takahashi I, Matsuzaki T, Kuroki H, et al. Physiological Reloading Recovers Histologically Disuse Atrophy of the Articular Cartilage and Bone by Hindlimb Suspension in Rat Knee Joint. Cartilage 2021;13:1530S-9S. [Crossref] [PubMed]
- Eckstein F, Wirth W, Guermazi A, et al. Brief report: intraarticular sprifermin not only increases cartilage thickness, but also reduces cartilage loss: location-independent post hoc analysis using magnetic resonance imaging. Arthritis Rheumatol 2015;67:2916-22. [Crossref] [PubMed]
- Liphardt AM, Mündermann A, Koo S, et al. Vibration training intervention to maintain cartilage thickness and serum concentrations of cartilage oligometric matrix protein (COMP) during immobilization. Osteoarthritis Cartilage 2009;17:1598-603. [Crossref] [PubMed]
Cite this article as: Zhou Z, Cheng X, Yang F, Zhang Z, Liu K, Zhang X, Huang H, Wang J. Weightlessness damaged the ultrastructure of knee cartilage and quadriceps muscle, aggravated the degeneration of cartilage. Ann Joint 2024;9:37.