Postoperative healing and complications based on anterior cruciate ligament reconstruction graft type
Introduction
The anterior cruciate ligament (ACL) is the most commonly injured ligament in the knee, with an annual reported incidence in the United States (US) of 1 in 3,500 people (1). Following an ACL rupture, there is limited potential for healing due to the local environment within the knee (2). Despite a recent resurgence in ACL repairs being performed globally, the current gold standard treatment for an ACL rupture is to reconstruct the torn ligament using either autograft or allograft tissue in patients with instability and those returning to pivoting sports. In 2017 it was estimated that approximately 400,000 ACL reconstructions (ACLRs) were performed annually in the US, although this number has likely increased each subsequent year (1).
Common autografts include bone-patellar tendon-bone (BTB), hamstring (gracilis and/or semitendinosus), and quadriceps (with or without patellar bone). Multiple allografts are available including BTB, hamstring (semitendinosus), tibialis posterior, tibialis anterior, and Achilles tendon (with or without bone). Graft fixation is usually performed within the bone tunnels or on the adjacent cortex with a button or a post-washer device.
Tendons and ligaments share a similar composition of dense connective tissue composed primarily of type I collagen (90%) and type III collagen (10%), proteoglycans, and cells (3). However, tendons and ligaments also have unique properties required for the biomechanical stresses to which they are subjected. This is demonstrated by the precise composition and arrangement of their matrix molecules (4). On an ultrastructural basis, ligaments contain cells that are more metabolically active, with rounded nuclei and higher DNA content, contain more type III collagen, more proteoglycans, less total collagen, a different amount of nonreducible collagen cross-links, and a different distribution of collagen fibril diameters compared to tendons (5,6).
ACL rupture is a devastating injury to athletes of all levels. Following ACL rupture, only 50–65% of recreational athletes are able to return to the preinjury level of sport (7,8). For professional athletes, it was recently reported that amongst National Football League (NFL) athletes sustaining an ACL injury during their career, only 55.8% were able to return to play postinjury, and of these athletes, only 28.5% remained in the league for at least 3 years postinjury (9). Whether an athlete is able to successfully return to their preinjury level of play is significantly influenced by the ability of the reconstructed graft tissue to remodel and heal; a process termed ligamentization. It is often debated the ways in which graft selection and fixation may influence healing, complications, and failure rate following ACLR.
Therefore, the purpose of this review is to summarize the process of ligamentization and graft healing and to discuss how graft type influences the rate and types of complications, failures, and infections.
Ligamentization
In 1986, Amiel et al. (10) used a rabbit model to demonstrate that a tendon autograft used to replace an excised ACL goes through a continuous process of tissue transformation resulting in the transplanted tendon becoming composed of tissue very similar to that of a normal ACL. This process was termed “ligamentization”. Since then, this process has been studied in a variety of animal models (10-14).
The process of ligamentization has been categorized into three phases: early healing, proliferation, and maturation (10,15). During the early healing phase, an accumulation of host inflammatory cells, including neutrophils and macrophages (16), are recruited to the periphery of the graft and release various cytokines, such as interleukin-1 (IL-1), tumor necrosis factor-alpha (TNF-α), transforming growth factor-beta (TGF-β), and matrix-metalloproteinase-1,13 (MMP-1,13) (17,18). Ultimately, this increase in cell signaling leads to the digestion of collagen and infiltration of repopulating cells. At the same time, during the early healing phase the tendon graft undergoes avascular necrosis, most notably in its central portion (19). During the process of avascular necrosis, several cytokines are released and lead to a subsequent cascade of growth factors that guide future steps (16,20).
After the graft undergoes avascular necrosis, the graft enters the proliferation phase. This is highlighted by an increased expression of vascular endothelial growth factor (VEGF) leading to vascular ingrowth of the graft. It is theorized that new blood vessels originate from the synovium, infrapatellar fat pad, and the pseudo-ligamentum mucosum (4). Adequate revascularization allows the graft to be infiltrated with new cells and undergo matrix remodeling, which is necessary for successful graft healing (11,21).
Finally, the graft enters the maturation phase. As the name suggests, this phase is characterized by continuous remodeling and maturation of the newly infiltrated cells into cells that closely resemble that of an intact ACL (22).
Based on evidence from animal studies, the time frame for these phases has been defined as the early healing occurring from the time of graft implantation through the 4th postoperative week, the proliferative phase occurring from postoperative week 4–10, and the maturation phase occurring as a continuous process beyond 10 weeks postoperatively, acquiring similar morphology and histologic appearance by 6 to 12 months (22-26), The results of these animals studies also suggest that biomechanical strength of the graft tissue varies greatly throughout the process of ligamentization, with a sharp decline in strength occurring during the early healing and proliferation phase, followed by a gradual return in strength seen throughout the maturation phase (Figure 1) (27).
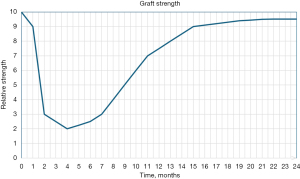
Graft biopsy
The relatively scarce data on graft healing in humans highlight substantial differences in the timeline of these phases in humans. Rougraff et al. (28) obtained graft biopsies in 23 subjects with previous ACLR using patellar tendon autografts. Biopsy was performed between 3 weeks to 6.5 years postoperatively. Histologic analysis of the biopsy specimens revealed the early healing, or “repopulation” phase occurred over the first 2 months postoperatively, with one biopsy obtained at 3 weeks postoperatively displaying a viable graft evidenced by increasing fibroblast number and active nuclear morphology. Interestingly, they did not find evidence of complete graft necrosis during this stage as previously described in animal studies. The process of proliferation then occurred over the next 10 months. During this phase, they noted a marked increase in the number of fibroblasts, active nuclear morphology, and neovascularity. Additionally, there were more areas of degeneration as the percentage of mature collagen decreased. The final phase of maturation occurred over the next 2 years, with grafts meeting histologic criteria of being “ligamentous” by 3 years (Figure 2A).
Abe et al. (29) performed light and electron microscopy analysis of biopsy specimens obtained from 21 knees previously reconstructed with a patellar tendon autograft. Biopsy was obtained at second look arthroscopy from 6 weeks to 15 months postoperatively. Their findings correlate with the early healing phase occurring over the first 5 months, the proliferative phase occurring from 5 to 9 months, and the maturation phase occurring beyond 9 months. They highlight in their findings that although at around 1 year postoperatively, the grafts were very similar in appearance to the native ACL on arthroscopic and light microscopic analysis, ultrastructural study suggests the grafts are still immature (Figure 2B).
Falconiero et al. (31) described similar findings in their study of hamstring (n=8) and patellar tendon (n=35) autografts. They found that collagen fiber alignment was restored around 6 months postoperatively; however, based on histologic analysis, full maturation was not achieved before 12 months postoperatively.
In their biopsy study of 37 hamstring autograft ACLRs, Sánchez et al. (30) observed the process of early healing occurred between 6 to 12 months postoperatively, proliferation from 13 to 18 months, and maturation from 19 to 24 months. In their study, by 24 months, specimens were histologically similar to the native ACL and characterized by the orientation of cells arranged in columns within the mature collagen matrix (Figure 2C).
Among the studies analyzing the ultrastructural appearance of both autograft and allograft tissue, an endpoint to the process of ligamentization has not been observed. The final ultrastructural appearance of the sampled graft tissue has commonly displayed small diameter, unimodal collagen fibril distribution, in contrast to the native ACL and hamstring tendon (HT) which consist of large diameter, bimodal collagen fibril distribution (29,32-35).
Graft healing within tunnels
Another consideration regarding graft healing is the incorporation of the graft within the bone tunnels. This differs depending on the graft type used. Bone-to-bone healing is seen in grafts that possess a bone block flanking the tendon, such as BTB grafts and quadriceps tendon (QT) grafts. Bone block healing within tunnels has been observed to resemble that of fracture healing, although somewhat more complex. Complete incorporation of the bone block in a round bone tunnel has been reported at 16 weeks postoperatively (36-38). Tendon-to-bone healing occurs through a separate process. This process is highlighted by the formation of fibrovascular tissue and Sharpey’s fibers at the graft-bone interface. This fibrovascular tissue then mineralizes, followed by mineralization of the entire tendon tissue within the tunnel. The presence of Sharpey’s fiber at the graft-bone interface is a marker of tendon cicatrization and has been observed beginning around 6 weeks postoperatively. Ultimately, the process of graft integration in the bone tunnel continues for 6–12 months (36,37).
Based on the results from basic science and animal studies, it is a widely held belief that bone-to-bone healing results in a quicker and more robust healing process (37,39-42). As a result of this belief, patients receiving a BTB autograft often are allowed to return to sport earlier than patients receiving a HT graft (43). However, there is a paucity of literature on rates of bone-to-bone vs. tendon-to-bone healing in human subjects to support this belief. To investigate this, Irvine et al. (44) embedded 0.8 mm tantalum beads into both HT (semitendinosus/gracilis) and BTB autografts in order to measure longitudinal motion of the grafts under various physiologic conditions using dynamic stereo X-rays. Round bone tunnels were created in both study conditions. Longitudinal motion was used a surrogate for graft healing and suspensory fixation was used in all cases. They found no difference in tibial tunnel motion or femoral tunnel motion between the HT and BTB patients at both 6 weeks and 1 year postoperatively. These results question the practice of allowing earlier return to play for patients receiving BTB grafts.
Complications
Graft failure/reinjury
A significant proportion of patients undergoing ACLR will experience a graft failure. Graft failure rates up to 40% have been reported in young, high risk patient populations (45). Failure of the ACL graft is multifactorial and can be associated with traumatic injury, incomplete or poor biologic healing, insufficient or inappropriate rehabilitation, and surgical technique (46,47). Choice of graft type has important clinical implications regarding failure risk among different patient populations.
Systematic reviews examining outcomes of adult populations undergoing ACLR have consistently found no difference in rate of failure between autograft and allograft reconstructions (48-50). However, data from the Multicenter Orthopaedic Outcomes Network (MOON), capturing a prospective longitudinal cohort of ACLR outcomes showed that the odds of a graft rupture after an allograft reconstruction were 4 times higher compared to autograft reconstructions (51). Similarly, a recent review examining failure rates in patients under the age of 19 years of age found a significantly higher rate of failure for allografts (25.5%) compared to autograft (8.5% for BTB; 16.6% for HT), with a near 4-fold increased risk of failure in patients receiving an allograft (52).
In a systematic review and meta-analysis evaluating outcomes of QT autograft (including with and without patellar bone block) vs. BTB autograft and HT autograft (including semitendinosus/gracilis or semitendinosus alone, 3 or 4 strands), there was no difference in failure rate between the three autografts (53). Similarly, results from meta-analyses by Mohtadi et al. (54), Xie et al. (55), and Samuelsen et al. (56) showed similar rates of failure between BTB autograft and HT autograft. A systematic review from the Scandinavian Knee Ligament Registry evaluated outcomes following 45,998 ACLR and found the rate of revision was reduced by 37% with use of BTB autograft compared to HT autograft, with HT autograft resulting in a 4-fold increase in risk of revision (57). Lind et al. evaluated the results of 16,579 ACLR from the Danish Knee Ligament Reconstruction Registry which showed revision rates were highest in patients receiving a QT autograft (4.7%) vs. HT autograft (2.3%) and BTB (1.5%) autograft (58). Of the patients receiving QT autograft, 54% included a patellar bone block. When patients were stratified by age, there were increased rates of revision amongst younger, higher activity level patients, with patients 16 to 20 years of age experiencing revision rates of 10.3% for QT autograft, 4.2% for BTB autograft, and 3.8% for HT autograft.
For women 25 years of age and younger, a systematic review evaluating outcomes following ACLR found use of BTB autograft was associated with a significantly lower risk of failure compared to HT autograft (6.1% and 17.4%, respectively) (59). Similarly, amongst younger patients, both male and female, there have been consistent results showing use of HT autograft is associated with higher rates of failure compared to BTB autograft with up to 5-year follow-up data (52,60-63). A study from the MOON knee group analyzing 6-year revision rates between BTB and HT autograft reconstruction among patients 14 to 22 years of age found that high-grade preoperative knee laxity, autograft type, and age were the 3 most influential predictors of graft revision, with rates of revision being 2.1 times higher in the HT autograft group compared to the BTB group (64).
Donor site morbidity
ACLR is an overall well tolerated procedure. However, morbidity from graft harvesting when using autologous tissue is clinically relevant. According to available literature, BTB autografts incur the greatest risk of donor site morbidity when compared to HT and QT autograft (65). Harvest site morbidity seen with BTB use includes anterior knee pain, sensory loss, patella fracture, patellar tendon tear/rupture, patellar tendinopathy, quadriceps amyotrophy, and flexion contracture (66). Anterior knee pain with kneeling has been reported in up to 31% of patients receiving BTB autograft (67). In a review by Cerulli et al. (65), reported rates of anterior knee pain ranged from 5% to 55% for BTB autografts. The incidence of patella fracture following BTB autograft harvesting is rare, with reported rates between 0.2% to 2.3% (68,69). Similarly, patella tendon tear is infrequently seen. The results of a database of 5,364 ACLR using BTB autograft found an incidence of patella tendon injury to be 0.2% (70). One report found an incidence of extension deficit >5° of 2% for BTB autograft ACLR (71).
Anterior knee pain is also associated with HT autograft use, but at a much lower incidence (54,65,67). Other complications that are more frequently seen in HT autografts include greater residual laxity, persistent flexion contracture, and knee flexion weakness (54).
In a prospective study of 958 cases, Rousseau et al. reported their rate of complications following ACLR using BTB and HT autografts (7). Their results showed a significant difference in the incidence of anterior knee pain during the 2-year follow-up period for BTB and HT grafts (23.3% vs. 12.6%, P<0.001). BTB autograft was also associated with a significantly higher rate of contralateral ACL rupture (5% vs. 2%, P=0.016). Meanwhile, HT autografts were associated with a significantly higher rate of pain around the fixation material (0.8% vs. 13.9%, P<0.001) and graft re-rupture (3.1% vs. 7%, P=0.023). Other reported complications did not show a significant difference between groups and included persistent pain at 2 years (3.1% vs. 2.5%), extension deficit (6.6% vs. 10%), secondary meniscal lesion (5% vs. 8.3%), fracture of the patella (1.1% vs. 0%), and other general complications including infection and thromboembolism (7% vs. 4.8%). Of note, regarding patella fractures, there were only three patients that experienced a patella fracture and all were following a BTB autograft.
Compared to BTB and HT, results following QT autograft have not been as extensively published. In a systematic review comparing results of ACLR with QT autograft vs. BTB and HT autograft, respectively, Mouarbes et al. (53) found QT autograft was associated with lower rates of anterior knee pain compared to BTB and better functional outcome scores compared to HT autograft. Other reported complications include quadriceps weakness, patella fracture (when a bone plug has been harvested), and sensory changes (72,73).
Infection
Infection following ACLR is an infrequent, yet devasting complication that can result in multiple reoperations, prolonged antibiotic therapy, graft removal and delayed revision reconstruction (74), and overall worse outcomes due to articular cartilage destruction and arthrofibrosis (75-78). The reported incidence of infection following ACLR is 0.14% to 1.7% (74,79).
It was previously believed that use of allograft tissue was associated with a higher incidence of infection, with evidence to support this (80-82). However, more recent evidence has failed to find a significant difference in infection rates between allograft and autograft reconstructions (79,80,83-85).
There have been a number of studies demonstrating use of HT autograft is associated with a higher incidence of infection compared to BTB autograft (80,83,86-88). In a large single institution cohort study, compared to BTB autograft, HT autograft and allograft were both independently associated with an increased risk of infection (odds ratio =4.39 and 5.27, respectively) (88). In another retrospective review, the use of HT autograft was associated with a 8.2 times higher risk of infection compared to BTB autograft (85). A recent meta-analysis comparing the rates of infection between BTB and HT autografts analyzed the results of 21 studies which demonstrated BTB autograft was associated with 77% lower incidence of infection compared to HT autograft. As a secondary measure, this study also found no significant difference in incidence of infection between autograft and allograft reconstructions (74).
Limited evidence is available citing the incidence of infection with QT autograft. A review of 17 studies using bone-QT autografts demonstrated a pooled incidence of infection of 1.5% (89). However, to our knowledge there currently are no existing meta-analyses comparing the incidence of infection between QT and BTB and/or HT.
Conclusions
Following reconstruction of the ACL, graft healing requires the tendon graft to undergo a continuous transformation until it is remodeled into tissue very similar to that of the native ACL, a process termed ligamentization. Evidence from human biopsy samples suggest that this process may continue for 1–3 years following ACLR.
The implication of incomplete graft healing prior to return to activity is the increased potential for graft failure. Based on prospective longitudinal cohort data, there are higher rates of graft failure following allograft reconstruction vs. autograft reconstruction. Amongst the autograft choices, similar rates of failure have been demonstrated for bone-patella tendon-bone, HT, and QT autografts in the general population. However, evidence from the Scandinvian and Danish registries suggest there may be higher rates of failure with QT autografts compared to bone-patella tendon-bone and HT autografts. Although similar rates of failure have been reported between bone-patella tendon-bone and HT autografts in the general population, use of HT autograft is associated with higher failure rates in younger, athletic patient populations.
Complications and infection following ACLR are infrequently seen. The most common complications include anterior knee pain, seen most frequently following BTB autograft, sensory changes, stiffness, weakness, and laxity. There is not compelling evidence to suggest allograft reconstruction carries an increased risk of infection compared to autograft reconstruction. However, there is evidence to suggest use of hamstring autograft carries a higher risk of infection compared to BTB autograft.
Acknowledgments
Funding: None.
Footnote
Peer Review File: Available at https://aoj.amegroups.com/article/view/10.21037/aoj-24-3/prf
Conflicts of Interest: All authors have completed the ICMJE uniform disclosure form (available at https://aoj.amegroups.com/article/view/10.21037/aoj-24-3/coif). A.G.G. serves as the infographics editor for the Arthroscopy journal and serves on the editorial board. He is a member of the AOSSM Council of Delegates. He is a paid consultant with Smith & Nephew and has received speaking fees from Ossur. The other authors have no conflicts of interest to declare.
Ethical Statement: The authors are accountable for all aspects of the work in ensuring that questions related to the accuracy or integrity of any part of the work are appropriately investigated and resolved.
Open Access Statement: This is an Open Access article distributed in accordance with the Creative Commons Attribution-NonCommercial-NoDerivs 4.0 International License (CC BY-NC-ND 4.0), which permits the non-commercial replication and distribution of the article with the strict proviso that no changes or edits are made and the original work is properly cited (including links to both the formal publication through the relevant DOI and the license). See: https://creativecommons.org/licenses/by-nc-nd/4.0/.
References
- Kaeding CC, Léger-St-Jean B, Magnussen RA. Epidemiology and Diagnosis of Anterior Cruciate Ligament Injuries. Clin Sports Med 2017;36:1-8. [Crossref] [PubMed]
- Murray MM, Fleming BC. Biology of anterior cruciate ligament injury and repair: Kappa delta ann doner vaughn award paper 2013. J Orthop Res 2013;31:1501-6. [Crossref] [PubMed]
- Siegel L, Vandenakker-Albanese C, Siegel D. Anterior cruciate ligament injuries: anatomy, physiology, biomechanics, and management. Clin J Sport Med 2012;22:349-55. [Crossref] [PubMed]
- Claes S, Verdonk P, Forsyth R, et al. The “ligamentization” process in anterior cruciate ligament reconstruction: what happens to the human graft? A systematic review of the literature. Am J Sports Med 2011;39:2476-83. [Crossref] [PubMed]
- Fujii K, Yamagishi T, Nagafuchi T, et al. Biochemical properties of collagen from ligaments and periarticular tendons of the human knee. Knee Surg Sports Traumatol Arthrosc 1994;2:229-33. [Crossref] [PubMed]
- Rumian AP, Wallace AL, Birch HL. Tendons and ligaments are anatomically distinct but overlap in molecular and morphological features--a comparative study in an ovine model. J Orthop Res 2007;25:458-64. [Crossref] [PubMed]
- Rousseau R, Labruyere C, Kajetanek C, et al. Complications After Anterior Cruciate Ligament Reconstruction and Their Relation to the Type of Graft: A Prospective Study of 958 Cases. Am J Sports Med 2019;47:2543-9. [Crossref] [PubMed]
- Sonnery-Cottet B, Saithna A. Editorial Commentary: Let’s ALL Agree-Anterior Cruciate Ligament Reconstruction Outcomes Need to Be Improved and Extra-Articular Procedures Have an Essential Role. Arthroscopy 2020;36:1702-5. [Crossref] [PubMed]
- Mody KS, Fletcher AN, Akoh CC, et al. Return to Play and Performance After Anterior Cruciate Ligament Reconstruction in National Football League Players. Orthop J Sports Med 2022;10:23259671221079637. [Crossref] [PubMed]
- Amiel D, Kleiner JB, Roux RD, et al. The phenomenon of “ligamentization”: anterior cruciate ligament reconstruction with autogenous patellar tendon. J Orthop Res 1986;4:162-72. [Crossref] [PubMed]
- Arnoczky SP, Tarvin GB, Marshall JL. Anterior cruciate ligament replacement using patellar tendon. An evaluation of graft revascularization in the dog. J Bone Joint Surg Am 1982;64:217-24. [Crossref] [PubMed]
- Bosch U, Kasperczyk WJ. Healing of the patellar tendon autograft after posterior cruciate ligament reconstruction--a process of ligamentization? An experimental study in a sheep model. Am J Sports Med 1992;20:558-66. [Crossref] [PubMed]
- Clancy WG Jr, Narechania RG, Rosenberg TD, et al. Anterior and posterior cruciate ligament reconstruction in rhesus monkeys. J Bone Joint Surg Am 1981;63:1270-84. [Crossref] [PubMed]
- Drez DJ Jr, DeLee J, Holden JP, et al. Anterior cruciate ligament reconstruction using bone-patellar tendon-bone allografts. A biological and biomechanical evaluation in goats. Am J Sports Med 1991;19:256-63. [Crossref] [PubMed]
- LaPrade RF, Geeslin AG, Murray IR, et al. Biologic Treatments for Sports Injuries II Think Tank-Current Concepts, Future Research, and Barriers to Advancement, Part 1: Biologics Overview, Ligament Injury, Tendinopathy. Am J Sports Med 2016;44:3270-83. [Crossref] [PubMed]
- Kawamura S, Ying L, Kim HJ, et al. Macrophages accumulate in the early phase of tendon-bone healing. J Orthop Res 2005;23:1425-32. [Crossref] [PubMed]
- Harkey MS, Luc BA, Golightly YM, et al. Osteoarthritis-related biomarkers following anterior cruciate ligament injury and reconstruction: a systematic review. Osteoarthritis Cartilage 2015;23:1-12. [Crossref] [PubMed]
- Xie X, Wu H, Zhao S, et al. The effect of platelet-rich plasma on patterns of gene expression in a dog model of anterior cruciate ligament reconstruction. J Surg Res 2013;180:80-8. [Crossref] [PubMed]
- Amiel D, Kleiner JB, Akeson WH. The natural history of the anterior cruciate ligament autograft of patellar tendon origin. Am J Sports Med 1986;14:449-62. [Crossref] [PubMed]
- Kuroda R, Kurosaka M, Yoshiya S, et al. Localization of growth factors in the reconstructed anterior cruciate ligament: immunohistological study in dogs. Knee Surg Sports Traumatol Arthrosc 2000;8:120-6. [Crossref] [PubMed]
- Kleiner JB, Amiel D, Roux RD, et al. Origin of replacement cells for the anterior cruciate ligament autograft. J Orthop Res 1986;4:466-74. [Crossref] [PubMed]
- Scheffler SU, Unterhauser FN, Weiler A. Graft remodeling and ligamentization after cruciate ligament reconstruction. Knee Surg Sports Traumatol Arthrosc 2008;16:834-42. [Crossref] [PubMed]
- Lui PP, Wong OT, Lee YW. Application of tendon-derived stem cell sheet for the promotion of graft healing in anterior cruciate ligament reconstruction. Am J Sports Med 2014;42:681-9. [Crossref] [PubMed]
- Sun Y, Chen W, Hao Y, et al. Stem Cell-Conditioned Medium Promotes Graft Remodeling of Midsubstance and Intratunnel Incorporation After Anterior Cruciate Ligament Reconstruction in a Rat Model. Am J Sports Med 2019;47:2327-37. [Crossref] [PubMed]
- Wei X, Mao Z, Hou Y, et al. Local administration of TGFβ-1/VEGF165 gene-transduced bone mesenchymal stem cells for Achilles allograft replacement of the anterior cruciate ligament in rabbits. Biochem Biophys Res Commun 2011;406:204-10. [Crossref] [PubMed]
- Lu CC, Ho CJ, Huang HT, et al. Effect of Freshly Isolated Bone Marrow Mononuclear Cells and Cultured Bone Marrow Stromal Cells in Graft Cell Repopulation and Tendon-Bone Healing after Allograft Anterior Cruciate Ligament Reconstruction. Int J Mol Sci 2021;22:2791. [Crossref] [PubMed]
- Wang HD, Li Z, Hu X, et al. Efficacy of Stem Cell Therapy for Tendon Graft Ligamentization After Anterior Cruciate Ligament Reconstruction: A Systematic Review. Orthop J Sports Med 2022;10:23259671221098363. [Crossref] [PubMed]
- Rougraff B, Shelbourne KD, Gerth PK, et al. Arthroscopic and histologic analysis of human patellar tendon autografts used for anterior cruciate ligament reconstruction. Am J Sports Med 1993;21:277-84. [Crossref] [PubMed]
- Abe S, Kurosaka M, Iguchi T, et al. Light and electron microscopic study of remodeling and maturation process in autogenous graft for anterior cruciate ligament reconstruction. Arthroscopy 1993;9:394-405. [Crossref] [PubMed]
- Sánchez M, Anitua E, Azofra J, et al. Ligamentization of tendon grafts treated with an endogenous preparation rich in growth factors: gross morphology and histology. Arthroscopy 2010;26:470-80. [Crossref] [PubMed]
- Falconiero RP, DiStefano VJ, Cook TM. Revascularization and ligamentization of autogenous anterior cruciate ligament grafts in humans. Arthroscopy 1998;14:197-205. [Crossref] [PubMed]
- Cho S, Muneta T, Ito S, et al. Electron microscopic evaluation of two-bundle anatomically reconstructed anterior cruciate ligament graft. J Orthop Sci 2004;9:296-301. [Crossref] [PubMed]
- Zaffagnini S, De Pasquale V, Marchesini Reggiani L, et al. Electron microscopy of the remodelling process in hamstring tendon used as ACL graft. Knee Surg Sports Traumatol Arthrosc 2010;18:1052-8. [Crossref] [PubMed]
- Dong S, Xie G, Zhang Y, et al. Ligamentization of Autogenous Hamstring Grafts After Anterior Cruciate Ligament Reconstruction: Midterm Versus Long-term Results. Am J Sports Med 2015;43:1908-17. [Crossref] [PubMed]
- Mayr HO, Stoehr A, Herberger KT, et al. Histomorphological Alterations of Human Anterior Cruciate Ligament Grafts During Mid-Term and Long-Term Remodeling. Orthop Surg 2021;13:314-20. [Crossref] [PubMed]
- Baxter FR, Bach JS, Detrez F, et al. Augmentation of bone tunnel healing in anterior cruciate ligament grafts: application of calcium phosphates and other materials. J Tissue Eng 2010;2010:712370. [Crossref] [PubMed]
- Fu FH, Bennett CH, Lattermann C, et al. Current trends in anterior cruciate ligament reconstruction. Part 1: Biology and biomechanics of reconstruction. Am J Sports Med 1999;27:821-30. [Crossref] [PubMed]
- Schiavone Panni A, Fabbriciani C, Delcogliano A, et al. Bone-ligament interaction in patellar tendon reconstruction of the ACL. Knee Surg Sports Traumatol Arthrosc 1993;1:4-8. [Crossref] [PubMed]
- Gulotta LV, Rodeo SA. Biology of autograft and allograft healing in anterior cruciate ligament reconstruction. Clin Sports Med 2007;26:509-24. [Crossref] [PubMed]
- Papageorgiou CD, Ma CB, Abramowitch SD, et al. A multidisciplinary study of the healing of an intraarticular anterior cruciate ligament graft in a goat model. Am J Sports Med 2001;29:620-6. [Crossref] [PubMed]
- Park MJ, Lee MC, Seong SC. A comparative study of the healing of tendon autograft and tendon-bone autograft using patellar tendon in rabbits. Int Orthop 2001;25:35-9. [Crossref] [PubMed]
- Tomita F, Yasuda K, Mikami S, et al. Comparisons of intraosseous graft healing between the doubled flexor tendon graft and the bone-patellar tendon-bone graft in anterior cruciate ligament reconstruction. Arthroscopy 2001;17:461-76. [Crossref] [PubMed]
- Mascarenhas R, Tranovich MJ, Kropf EJ, et al. Bone-patellar tendon-bone autograft versus hamstring autograft anterior cruciate ligament reconstruction in the young athlete: a retrospective matched analysis with 2-10 year follow-up. Knee Surg Sports Traumatol Arthrosc 2012;20:1520-7. [Crossref] [PubMed]
- Irvine JN, Arner JW, Thorhauer E, et al. Is There a Difference in Graft Motion for Bone-Tendon-Bone and Hamstring Autograft ACL Reconstruction at 6 Weeks and 1 Year? Am J Sports Med 2016;44:2599-607. [Crossref] [PubMed]
- Getgood AMJ, Bryant DM, Litchfield R, et al. Lateral Extra-articular Tenodesis Reduces Failure of Hamstring Tendon Autograft Anterior Cruciate Ligament Reconstruction: 2-Year Outcomes From the STABILITY Study Randomized Clinical Trial. Am J Sports Med 2020;48:285-97. [Crossref] [PubMed]
- Wiggins AJ, Grandhi RK, Schneider DK, et al. Risk of Secondary Injury in Younger Athletes After Anterior Cruciate Ligament Reconstruction: A Systematic Review and Meta-analysis. Am J Sports Med 2016;44:1861-76. [Crossref] [PubMed]
- Johnson DL, Swenson TM, Irrgang JJ, et al. Revision anterior cruciate ligament surgery: experience from Pittsburgh. Clin Orthop Relat Res 1996;100-9. [Crossref] [PubMed]
- Mariscalco MW, Magnussen RA, Mehta D, et al. Autograft versus nonirradiated allograft tissue for anterior cruciate ligament reconstruction: a systematic review. Am J Sports Med 2014;42:492-9. [Crossref] [PubMed]
- Cvetanovich GL, Mascarenhas R, Saccomanno MF, et al. Hamstring autograft versus soft-tissue allograft in anterior cruciate ligament reconstruction: a systematic review and meta-analysis of randomized controlled trials. Arthroscopy 2014;30:1616-24. [Crossref] [PubMed]
- Haybäck G, Raas C, Rosenberger R. Failure rates of common grafts used in ACL reconstructions: a systematic review of studies published in the last decade. Arch Orthop Trauma Surg 2022;142:3293-9. [Crossref] [PubMed]
- Kaeding CC, Aros B, Pedroza A, et al. Allograft Versus Autograft Anterior Cruciate Ligament Reconstruction: Predictors of Failure From a MOON Prospective Longitudinal Cohort. Sports Health 2011;3:73-81. [Crossref] [PubMed]
- Cruz AI Jr, Beck JJ, Ellington MD, et al. Failure Rates of Autograft and Allograft ACL Reconstruction in Patients 19 Years of Age and Younger: A Systematic Review and Meta-Analysis. JB JS Open Access 2020;5:e20.00106.
- Mouarbes D, Menetrey J, Marot V, et al. Anterior Cruciate Ligament Reconstruction: A Systematic Review and Meta-analysis of Outcomes for Quadriceps Tendon Autograft Versus Bone-Patellar Tendon-Bone and Hamstring-Tendon Autografts. Am J Sports Med 2019;47:3531-40. [Crossref] [PubMed]
- Mohtadi NG, Chan DS, Dainty KN, et al. Patellar tendon versus hamstring tendon autograft for anterior cruciate ligament rupture in adults. Cochrane Database Syst Rev 2011;2011:CD005960. [Crossref] [PubMed]
- Xie X, Liu X, Chen Z, et al. A meta-analysis of bone-patellar tendon-bone autograft versus four-strand hamstring tendon autograft for anterior cruciate ligament reconstruction. Knee 2015;22:100-10. [Crossref] [PubMed]
- Samuelsen BT, Webster KE, Johnson NR, et al. Hamstring Autograft versus Patellar Tendon Autograft for ACL Reconstruction: Is There a Difference in Graft Failure Rate? A Meta-analysis of 47,613 Patients. Clin Orthop Relat Res 2017;475:2459-68. [Crossref] [PubMed]
- Hamrin Senorski E, Svantesson E, Baldari A, et al. Factors that affect patient reported outcome after anterior cruciate ligament reconstruction-a systematic review of the Scandinavian knee ligament registers. Br J Sports Med 2019;53:410-7. [Crossref] [PubMed]
- Lind M, Strauss MJ, Nielsen T, et al. Quadriceps tendon autograft for anterior cruciate ligament reconstruction is associated with high revision rates: results from the Danish Knee Ligament Registry. Knee Surg Sports Traumatol Arthrosc 2020;28:2163-9. [Crossref] [PubMed]
- Etzel CM, Nadeem M, Gao B, et al. Graft Choice for Anterior Cruciate Ligament Reconstruction in Women Aged 25 Years and Younger: A Systematic Review. Sports Health 2022;14:829-41. [Crossref] [PubMed]
- Kim SJ, Choi CH, Kim SH, et al. Bone-patellar tendon-bone autograft could be recommended as a superior graft to hamstring autograft for ACL reconstruction in patients with generalized joint laxity: 2- and 5-year follow-up study. Knee Surg Sports Traumatol Arthrosc 2018;26:2568-79. [Crossref] [PubMed]
- Kitchen BT, Mitchell BC, Cognetti DJ, et al. Outcomes After Hamstring ACL Reconstruction With Suture Tape Reinforcement in Adolescent Athletes. Orthop J Sports Med 2022;10:23259671221085577. [Crossref] [PubMed]
- Liukkonen RJ, Ponkilainen VT, Reito A. Revision Rates After Primary ACL Reconstruction Performed Between 1969 and 2018: A Systematic Review and Metaregression Analysis. Orthop J Sports Med 2022;10:23259671221110191. [Crossref] [PubMed]
- Rahardja R, Zhu M, Love H, et al. Effect of Graft Choice on Revision and Contralateral Anterior Cruciate Ligament Reconstruction: Results From the New Zealand ACL Registry. Am J Sports Med 2020;48:63-9. [Crossref] [PubMed]
- MOON Knee Group. Anterior Cruciate Ligament Reconstruction in High School and College-Aged Athletes: Does Autograft Choice Influence Anterior Cruciate Ligament Revision Rates? Am J Sports Med 2020;48:298-309. [Crossref] [PubMed]
- Cerulli G, Placella G, Sebastiani E, et al. ACL Reconstruction: Choosing the Graft. Joints 2013;1:18-24. [PubMed]
- Thaunat M, Fayard JM, Sonnery-Cottet B. Hamstring tendons or bone-patellar tendon-bone graft for anterior cruciate ligament reconstruction? Orthop Traumatol Surg Res 2019;105:S89-94. [Crossref] [PubMed]
- Corry IS, Webb JM, Clingeleffer AJ, et al. Arthroscopic reconstruction of the anterior cruciate ligament. A comparison of patellar tendon autograft and four-strand hamstring tendon autograft. Am J Sports Med 1999;27:444-54. [Crossref] [PubMed]
- Piva SR, Childs JD, Klucinec BM, et al. Patella fracture during rehabilitation after bone-patellar tendon-bone anterior cruciate ligament reconstruction: 2 case reports. J Orthop Sports Phys Ther 2009;39:278-86. [Crossref] [PubMed]
- Lee GH, McCulloch P, Cole BJ, et al. The incidence of acute patellar tendon harvest complications for anterior cruciate ligament reconstruction. Arthroscopy 2008;24:162-6. [Crossref] [PubMed]
- Benner RW, Shelbourne KD, Urch SE, et al. Tear patterns, surgical repair, and clinical outcomes of patellar tendon ruptures after anterior cruciate ligament reconstruction with a bone-patellar tendon-bone autograft. Am J Sports Med 2012;40:1834-41. [Crossref] [PubMed]
- Freedman KB, D’Amato MJ, Nedeff DD, et al. Arthroscopic anterior cruciate ligament reconstruction: a metaanalysis comparing patellar tendon and hamstring tendon autografts. Am J Sports Med 2003;31:2-11. [Crossref] [PubMed]
- Mulford JS, Hutchinson SE, Hang JR. Outcomes for primary anterior cruciate reconstruction with the quadriceps autograft: a systematic review. Knee Surg Sports Traumatol Arthrosc 2013;21:1882-8. [Crossref] [PubMed]
- Slone HS, Romine SE, Premkumar A, et al. Quadriceps tendon autograft for anterior cruciate ligament reconstruction: a comprehensive review of current literature and systematic review of clinical results. Arthroscopy 2015;31:541-54. [Crossref] [PubMed]
- Bansal A, Lamplot JD, VandenBerg J, et al. Meta-analysis of the Risk of Infections After Anterior Cruciate Ligament Reconstruction by Graft Type. Am J Sports Med 2018;46:1500-8. [Crossref] [PubMed]
- Donatto KC. Orthopedic management of septic arthritis. Rheum Dis Clin North Am 1998;24:275-86. [Crossref] [PubMed]
- Goldenberg DL, Reed JI. Bacterial arthritis. N Engl J Med 1985;312:764-71. [Crossref] [PubMed]
- Hogan CJ, Fang GD, Scheld WM, et al. Inhibiting the inflammatory response in joint sepsis. Arthroscopy 2001;17:311-5. [Crossref] [PubMed]
- McAllister DR, Parker RD, Cooper AE, et al. Outcomes of postoperative septic arthritis after anterior cruciate ligament reconstruction. Am J Sports Med 1999;27:562-70. [Crossref] [PubMed]
- Indelli PF, Dillingham M, Fanton G, et al. Septic arthritis in postoperative anterior cruciate ligament reconstruction. Clin Orthop Relat Res 2002;182-8. [Crossref] [PubMed]
- Brophy RH, Wright RW, Huston LJ, et al. Factors associated with infection following anterior cruciate ligament reconstruction. J Bone Joint Surg Am 2015;97:450-4. [Crossref] [PubMed]
- Wang C, Ao Y, Wang J, et al. Septic arthritis after arthroscopic anterior cruciate ligament reconstruction: a retrospective analysis of incidence, presentation, treatment, and cause. Arthroscopy 2009;25:243-9. [Crossref] [PubMed]
- Crawford C, Kainer M, Jernigan D, et al. Investigation of postoperative allograft-associated infection in patients who underwent musculoskeletal allograft implantation. Clin Infect Dis 2005;41:195-200. [Crossref] [PubMed]
- Barker JU, Drakos MC, Maak TG, et al. Effect of graft selection on the incidence of postoperative infection in anterior cruciate ligament reconstruction. Am J Sports Med 2010;38:281-6. [Crossref] [PubMed]
- Katz LM, Battaglia TC, Patino P, et al. A retrospective comparison of the incidence of bacterial infection following anterior cruciate ligament reconstruction with autograft versus allograft. Arthroscopy 2008;24:1330-5. [Crossref] [PubMed]
- Maletis GB, Inacio MC, Reynolds S, et al. Incidence of postoperative anterior cruciate ligament reconstruction infections: graft choice makes a difference. Am J Sports Med 2013;41:1780-5. [Crossref] [PubMed]
- Judd D, Bottoni C, Kim D, et al. Infections following arthroscopic anterior cruciate ligament reconstruction. Arthroscopy 2006;22:375-84. [Crossref] [PubMed]
- Geib TM, Shelton WR, Phelps RA, et al. Anterior cruciate ligament reconstruction using quadriceps tendon autograft: intermediate-term outcome. Arthroscopy 2009;25:1408-14. [Crossref] [PubMed]
- Marom N, Kapadia M, Nguyen JT, et al. Factors Associated With an Intra-articular Infection After Anterior Cruciate Ligament Reconstruction: A Large Single-Institution Cohort Study. Am J Sports Med 2022;50:1229-36. [Crossref] [PubMed]
- Singh H, Glassman I, Sheean A, et al. Less than 1% risk of donor-site quadriceps tendon rupture post-ACL reconstruction with quadriceps tendon autograft: a systematic review. Knee Surg Sports Traumatol Arthrosc 2023;31:572-85. [Crossref] [PubMed]
Cite this article as: Boyd E, Endres NK, Geeslin AG. Postoperative healing and complications based on anterior cruciate ligament reconstruction graft type. Ann Joint 2024;9:30.