Connexin 43 in the function and homeostasis of osteocytes: a narrative review
Introduction
Bone tissue consists of three main cell types: osteocytes, osteoblasts, and osteoclasts. Osteocytes are the most abundant cell type in bone and play an important role in mediating the mechanical sensitivity of bone and maintaining homeostasis by regulating the activities of osteoclasts and osteoblasts (1,2). They are embedded inside the bone mineral matrix, and long dendritic structures of osteocytes form a network connecting neighboring osteocytes and other cells, such as osteoblasts and osteoclasts, on the bone surface (3). Mechanical stress induces the formation and remodeling of bone tissue via cell-to-cell contact to achieve bone tissue reconstruction (4). The mechanisms of intercellular communication include chemical signal transmission, synaptic transmission, and gap junction (GJ) communication. Among them, the GJ channels play an important role in the process of converting mechanical signals into biological effects (5,6).
In bones, intercellular information transmission is mainly accomplished by GJ channels. The GJ channel is a membrane protein structure commonly existing between adjacent cells, which consists of a GJ protein called connexin (Cx) (6). Through the GJ channels, the cells in bone permit the exchange of small molecules, such as ions, metabolites, and signal molecules (7). The GJ channel composed of connexin 43 (Cx43) usually allows molecules of <1.2 kDa to pass through and typically limits larger molecules including proteins and nucleic acids from passing through (8,9). The basic structure of the GJ is the unit protein, where six connexins aggregate and surround each other to form a hydrophilic pore channel with a diameter of about 1.5 to 2 nm. This structure is called a “hemichannel”, two of which penetrate through the adjacent cell membrane (10). The “hemichannels” are anchored and docked with each other to form a full channel, namely the GJ. The openings at both ends of the GJ channel are located in the cytoplasm of two adjacent cells, which will be direct channels for cell-to-cell material exchange. The type of Cx determines the dimension of the GJ channel. GJ-mediated intercellular signal transduction plays an important role in tissue growth and development, maintaining the stability of the intracellular environment, cell differentiation, and tumorigenesis (11,12). Recently, Cx43 was found to be the most abundantly expressed Cx in human bone and cartilage and plays an important role in maintaining the functional homeostasis of cartilage. In addition to the classic GJ intercellular communication (GJIC), there are also barrier-free interstitial junctions in the membrane that act as direct conduits between the cytoplasm and the extracellular environment (13,14). The ability of GJIC to transmit biophysics to the entire network of bone cells and osteoblasts suggests that GJIC may be an important mechanism by which the mechanical forces are transmitted through bone and subsequently lead to bone formation. In addition to bone formation, GJIC also regulates bone absorption (5,15). Cx43 is also critical for bone homeostasis, regulating bone cell function by actively participating in the regulation of signals, gene expression, cell survival, and the ability to respond to cues such as mechanical stress (16). Cx43 and macrophage functions are closely intertwined, including interactions with neighboring cells, microenvironment, migration to sites of inflammation, antigen presentation, and immune regulation (17). Furthermore, numerous studies have found frequent aberrant expression of Cx43 in myocardial infarction, diabetes, and tumors (18-24). In this study, we review the mechanism of Cx43’s role in the mechanical stimulation and signal transduction of osteocytes to provide theoretical support for new molecular markers and novel targets for the treatment of bone diseases. Numerous studies have confirmed the role of Cx43 in osteoblast function and homeostasis. A better understanding of the pathways by which Cx43 regulates bone tissue remodelling may assist in the development of therapeutic approaches aimed at treating disorders of the human skeletal system. We present this article in accordance with the Narrative Review reporting checklist (available at https://aoj.amegroups.com/article/view/10.21037/aoj-23-65/rc).
Methods
We performed a narrative review of the literature in the PubMed, EMBASE, Cochrane Library, and Web of Science databases to find manuscripts published through June 2023 on the role of Cx43 in the function and homeostasis of osteocytes. The following search terms were used in this review: “connexin 43” or “Cx43” and “Osteocytes” [Mesh]. Two authors searched the databases independently, and a third reviewer mediated any disagreements in the results of the two screeners. Peer-reviewed, published literature, including narrative review papers, was included. Studies, editorials, letters to the editor, and abstracts were to be excluded when they involved animals. The reference lists of included papers were searched manually and included if they met the inclusion criteria. The search strategy is summarized in Table 1.
Table 1
Items | Specification |
---|---|
Date of search | June 2023 |
Databases and other sources searched | PubMed, EMBASE, Cochrane Library, and Web of Science |
Search terms used | “Connexin 43” OR “Cx43” AND “Osteocytes” [Mesh] |
Timeframe | From 1970 to June 2023 |
Inclusion and exclusion criteria | Inclusion criteria: (I) English-language papers; (II) studies evaluating connexin 43 and osteocytes function and homeostasis; (III) peer-reviewed, published literature, including narrative review papers. Exclusion criteria: (I) publications with duplications or studies with overlapping data from the same author; (II) abstracts, case reports, proceedings, letters, reviews, and meta-analyses; (III) incomplete outcome data |
Selection process | Two authors searched the database independently. A third reviewer mediated any disagreements between the two researchers |
Discussion
Osteocyte Cx43 and mechanical stimulation
Osteocyte Cx43 and mechanical loading
The location of osteocytes in bone makes them a prime candidate for mechanosensory cells in bone tissue. The application of mechanical strain in bone cells leads to the redistribution of Cx43, the assembly of GJ channels, and the formation of other functional GJs (25-27). The expression and localization of Cx43 and the formation of interstitial connections in bone cells can be regulated by mechanical stimulation. The interstitial junction remains important in the response of bone cells to mechanical stimulation and the subsequent increase in osteoblast differentiation. Previous studies have shown that hemichannels formed by Cx43 are highly formed in bone cells and mechanically sensitive (28,29). Cx43 plays an indispensable role in maintaining the bone homeostasis and activity of mature osteocytes (5,30,31).
Bone tissue is remodeled under mechanical stress. The most abundant bone cells are considered stress receptors. Previous studies have shown that antibodies produced using the Cx43 prostaglandin E2 (PGE2) ring as an antigen can block dye absorption observed after mechanical stimulation (32,33). Studies have also indicated that the Cx43 hemichannel is the main mechanosensory channel expressed in bone cells (34). Cx43 is highly expressed in osteocytes, which are ideally positioned to sense and transmit mechanical force-induced signals in bone and osteoblasts. The expression of Cx43 is enhanced by loading in cultured osteoblasts and osteocytes in vivo (35). Cx43 involvement in controlling osteocyte function and ultimate bone mass is complex and varies depending on the effect. For example, the loss of Cx43 differentially modulates the response of bone to mechanical load on the surface of the periosteum and endosporium (36,37). Inhibition of osteoblast Cx43 channels promotes unloading-induced bone loss, which mainly occurs in the cortical regions; in addition, hemichannels protect osteoblasts from apoptosis and promote periosteal bone remodeling, while GJs regulate intracortical osteoclast activity in response to unloading (38,39).
However, the loss of Cx43 not only reduces the anabolic effects of mechanical load but also blunts the effects of bone loss due to mechanical load and even aging (40,41). This means that the signals transmitted by Cx43 may be anabolic or catabolic, depending on the environment, such as aging, mechanical loading or unloading, or even location (4,42). In addition, the survival effect of a lack of mechanical stimulation in normal off-bed conditions may account for the increased apoptosis of osteocytes observed in osteoblasts/osteocytes or mice lacking Cx43 in their osteocytes. Several studies have shown that through mechanical channel stimulation, the channel composed of Cx43 can produce interstitial junction communication, and fluid flow can increase Cx43 expression, phosphorylation, and plasma membrane localization (43-45).
In vitro, osteoblasts and osteocytes can respond to mechanical disturbances and/or mechanical stresses caused by fluid flow by generating calcium oscillations that can propagate from cell to cell in a Cx43-dependent manner (45,46). The Cx43-dependent calcium oscillation between osteocytes and osteoblasts can replicate in vitro in complete bone in response to mechanical stresses on the cells (47,48). Osteocytes sense mechanical stimuli and convert them into chemical signals that are transmitted to other bone cells and the extracellular environment through interstitial connections between adjacent cells or hemichannels between cells (49). In bone cells, Cx43 is the most abundant Cx responsible for the formation of interstitial junctions and hemichannels, thus playing a key role in mediating mechanical transduction. Mechanical stimulation affects the expression and cell distribution of Cx43 in expressed bone cells. The strain experienced at the tissue level under normal physiological load is insufficient to produce bone anabolic effects at the cellular level, suggesting that these forces are amplified as they propagate through the skeleton. The Piezoelectric effect and flow potential are two mechanisms of mechanical conduction (6,41,50).
In vitro studies have shown that GJIC makes bone cell networks sensitive to a variety of extracellular signals, including load induction signals. In addition, mechanical signals detected by bone cells can be communicated to osteoblasts through GJIC (51,52). Mechanical stimulation increases the expression and phosphorylation of Cx43 and Cx43-mediated GJIC. In addition to the role of GJs in the response of bone cells to mechanical loading, emerging evidence suggests that the Cx43-semicircle channel may also be a key component in osteogenic signal transduction in response to mechanical stimuli (53,54). Murine long bone osteocyte-Y4 (MLO-Y4) cells in a low-density culture showed enhanced hemichannel activity in response to fluid flow, which was attenuated by the inhibition of hemichannel activity or Cx43 expression (55). Cx43 has also been shown to play an important role in the ability of bone cells to induce biochemical responses to mechanical stress (56).
Osteocyte Cx43 and fluid shear stress
Osteocytes are the main mechanical sensory cells in bone, and fluid flow is the main type of mechanical stimulation to osteocytes (57,58). The Cx43 hemichannel in bone cells is highly sensitive to mechanical load (59). GJIC is also important for cellular mechanical transduction. Fluid shear stress and mechanical strain increase GJIC between bone cells (54,60). In this study, the loss of Cx43 in osteocytes and osteoblasts was shown to result in delayed osteoblast differentiation, reduced bone formation, and impaired mechanical properties (34). Cx43 plays an important role in restoring normal bone structure and mechanical strength by regulating β-catenin signal transduction during fracture healing (61). Fluid flow is thought to represent major biophysical signals in mechanical transduction, and several biological effects have been described following the application of fluid flow to osteoblasts (62). Shear stress caused by mechanical load promotes nutrient and bone regulator exchange and initiates biochemical reactions. Osteocytes are well positioned in the skeleton and can sense the magnitude of mechanical strain, which is critical to the bone’s adaptive response to load. Experimental studies have shown that osteocytes are sensitive to pressure applied to intact bone tissue and cell culture (63,64).
In bone cells, glycocalyx degradation has been shown to result in decreased PGE2 release after fluid shear stress (65). Mechanical loads play a crucial role in maintaining normal bone structure and function in bone tissue. Over the past decade or so, osteocytes have been recognized as modulating the biological responses of other osteocytes to mechanical loads (66). Previous studies have shown that bone cells respond to mechanical loading as well as the release of molecules such as PGE2 and nitric oxide (NO) (67-69). Since bone cells are surrounded by fluid-filled space, they are constantly subjected to the shear stress of fluid flow (70). The application of flow shear stress on osteoblastic MLO-Y4 cells increases the number and length of dendrites (71). Recently, mechanical loads in the form of fluid flow shear stress (FFSS) have been shown to mimic the flow of bone fluid in the lacrimal duct network of bone cells, thereby preventing glucocorticoid-induced apoptosis (72). The shear stress induced by fluid flow stimulates intercellular communication mediated by intercellular connectivity in osteoblast-like MLO-Y4 cells and increases the expression of Cx43 (73). This stimulation is further enhanced during the post-flow period. The report showed that fluid flow increases the release of PGE2 in osteoblast-like MLO-Y4 cells and that PGE2 is involved in the stimulation of intercellular communication by shear stress induced by fluid flow. These observations suggest that PGE2 is a factor that increases the interstitial junction activity of bone cells in response to shear stress after fluid flow (74).
Moreover, we recently demonstrated that FFSS induces the rapid opening of the hemichannel, which in turn mediates the release of PGE2 in osteolytic MLO-Y4 cells (74,75). A recent study showed that adenosine triphosphate (ATP) and PGE2 release oscillating fluid induced OCY454 osteocytes (76). In response to high and low shear stresses, Cx43 phosphorylation and reduced functional intercellular coupling also occur. Interestingly, the low shear stress increases the expression of Cx43 messenger RNA (mRNA). In contrast, the expression of Cx43 mRNA decreases under high shear stress, so the gap connections could be rearranged or formed according to shear stress (77). Fluid shear stress promotes the opening of mechanically-sensitive hemichannels in osteolytic MLO-Y4 cells, leading to the release of regulatory factors such as PGE2 and ATP (55). A study has shown that the shear stress reduces the expression of Cx43 protein in MLO-Y4 bone cells and reduced GJIC (77).
The expression of Cx43 decreases with the increase of shear stress. However, differences in Cx43 and Cx45 mRNA expression were observed between low shear strength and high shear strength. Cx43 mRNA increases under low shear stress but there is no change in Cx45 expression; meanwhile, Cx43 mRNA decreases under high shear stress, which is also accompanied by an increase in the mRNA expression of Cx45. These findings suggest that Cx43 may produce a compensatory response due to the inhibition of Cx43, and the composition of GJ can be altered in response to specific mechanical stimulation. Taken together, these studies suggest that Cx43 and GJIC are critical for the anabolic response of bone to mechanical load. The formation of gap connections can be regulated by fluid flow but there is also evidence that hemichannels play an important role in conducting mechanical loads. MLO-Y4 cells are cultured at very low densities, thus minimizing the contribution of GJIC and increasing its hemichannel activity in response to fluid flow (51). In bone cells, fluid shear stress induces the release of extracellular mechanical signaling molecules required for bone homeostasis, such as PGE2, NO, ATP, and vascular endothelial growth factor (VEGF) (78).
Osteocyte Cx43 and gravity
Several papers have reported on the expression of Cx43 in bone cells in response to gravity. A study in our laboratory has shown that the expression of Cx43 is reduced after low gravity and supergravity treatment provided by parabolic flight (79). Another study has also shown that intercellular communication significantly decreases after 24 hours of multi-dimensional gravity in MLO-Y4 cells (80). A Cx43 deficiency mouse model demonstrated that the bone is insensitive to hindlimb suspension (HLS) modeling of weightlessness (41,81,82). Similar results were shown after injecting botulinum toxin A (BtxA) to simulate mechanical unloading (83). Two studies reported that the conditioned deletion of Cx43 in osteoblasts and osteocytes partially resisted cortical bone loss caused by mechanical unloading (38,81), suggesting that Cx43 was involved in the skeletal response to weightless conditions.
Osteocyte Cx43 and signal transduction
Cx43 is involved in the intercellular transmission of second messengers and enhances the signaling pathways activated by these second messengers in adjacent cells, thus increasing the activation of signaling pathways downstream of these intercellular connections (Figure 1). The second mechanism through which Cx43 affects bone is its role in transmitting mechanical load signals between bone cells. Mechanical forces induce the formation of intercellular connections, and the ability of intercellular communication is consistent both in vitro and in vitro (Table 2). Previous experimental studies have shown that bone cells respond to fluid shear stress and release signaling molecules such as prostaglandins, NO, calcium ion (Ca2+), and other second messengers (84-87). It has also been shown that fluid-induced shear stress is an important biophysical signal in bone mechanical transduction. In osteoblasts and osteoblastic cell lines, the magnitude of fluid shear stress expected to occur in bone tissue disrupts connective communication and rearranges connective whites and to some extent the synthetic shear stresses of specific connective proteins. This disconnection from the osteocyte network due to fluid shear stress can provide a portion of the signal, thereby disconnecting the cell or the rest of the network to initiate focal bone remodeling (77).
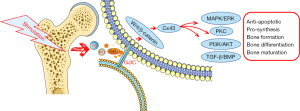
Table 2
Subject | Authors (brief title) | Results |
---|---|---|
Osteoblasts and Cx43 | Lilian I. Plotkin (27) (Cx43 and mechanotransduction in bone) | Cx43 participates in mechanotransduction |
Cx43 and bone | Lilian I. Plotkin (11) (Cx43 and bone: not just a gap junction protein) | Cx43 is responsible for signal transduction |
Gap junctions and hemichannels in osteocytes | Alayna E. Loiselle (6) (Gap junction and hemichannel functions in osteocytes) | Cx43 can mediate mechanical stimulation through gap junctions and hemichannels in bone cells, leading to bone formation or absorption |
CX43 affects bone homeostasis | Atum M. Buo (39) (Gap Junctional Regulation of Signal Transduction in Bone Cells) | Cx43 gap junctions and hemichannels regulate various molecular mechanisms in bone cells for cellular signaling pathways, gene expression, mechanical transduction, and cell survival |
GJIC regulates the formation, differentiation, survival, and apoptosis of osteoblasts | Nidhi Batra (7) (Gap Junctions and Hemichannels in Signal Transmission, Function, and Development of Bone) | The role of interstitial connections and hemichannels in regulating signal transduction, function and development of bone cells |
Gap junction and bone development | Joseph P. Stains (14) (Gap junctions in skeletal development and function) | Connexins play an important role in bone form and function |
Cx43 and osteoblasts | Joseph P. Stains (16) (Molecular mechanisms of osteoblast/osteocyte regulation by connexin43) | Cx43 regulates anabolic and catabolic responses in osteoblasts and osteocyte biology |
Cx43, Connexin 43; GJIC, gap junction intercellular communication.
In summary, there is growing evidence that Cx43 is a key component of intracellular mechanisms responsible for signaling in bone in response to pharmacological, hormonal, and mechanical stimuli. Under HO-induced oxidative stress, mitochondrial Cx43 (mtCx43) enhances mitochondrial ATP production to protect cells from oxidative stress by participating in the maintenance of the proton gradient between the membrane space and the matrix (88). This mechanism may be the result of small molecule release and/or the activation of other signaling pathways (6). In addition to their role in GJ channels or hemichannels, release proteins (including Cx43) regulate cellular behavior by interacting with intercellular signaling molecules (7). In addition to the Cx43 hemichannel, the role of the ATP receptor (ionic P2X7 receptor) in bone cell mechanical transduction and signaling has been proposed (89). The activation of P2X7 receptors (P2X7Rs) has been shown to mediate the ATP-induced release of certain cell types, and P2X7R deficiency eliminates PGE2 release from osteoblasts in response to fluid shear stress (90). Mechanical signal transduction represents another aspect of osteocyte regulation in which Cxplays a crucial role. GJs and hemichannels formed by Cx43 can facilitate communication connections between cells. Osteocytes can affect the activities of osteoblasts and osteoclasts through interstitial junctions (91), which are considered to be important components of extracellular signal transduction, integration, and amplification in bone. The signals transmitted by Cx43 may be anabolic or catabolic, depending on the environment, such as aging, mechanical loading or unloading, or even location (43). In osteocytes, the hemichannel is open and the intracellular signaling pathway that affects osteoblastic function is also regulated by Cx43 (6).
Multiple in vitro structural and functional studies have shown that the Cx43 c-terminal plays an important role in the signaling and function of bone cells. Importantly, the over-expression of Cx43 requires the c-terminal for signaling and transcription in bone cells but lacks the c-terminal to mimic the effect of Cx43 (92). It exhibits an antagonistic expression for signaling through the extracellular regulated kinase (ERK) and protein kinase C (PKC) pathways and osteoblast genes. This requirement for the c-terminal is consistent with the fact that PKC forms a complex with Cx43 through its c-terminal domain and, upon activation, transits from Cx43 to the nucleus interacting with runt-related transcription factor 2 (Runx2) (93). The researchers found that apoptotic osteoblasts trigger receptor activator of nuclear factor-κB ligand (RANKL) production in vivo by surviving neighboring (i.e., bystander) osteoblasts; however, the mechanism is unclear. They subsequently found that the apoptosis of MLOY-4 osteoblasts in a new multiscale fluidic device (the Macro-micro-nano, or Mµn) resulted in increased osteoblast RANKL expression in adjacent compartments (94). In addition, titanium particles can increase Cx43 expression in osteoblasts, and osteoblasts may be involved in regulating osteoclast function through Cx43 during the OPP process.
The wingless/integrated (Wnt)/β-catenin signaling pathway is an important signal regulation system in vivo. The Wnt signaling pathway is involved in the adaptive response of bone to stress stimulation. Following stress stimulation, bone cells can induce the rapid activation of their internal Wnt signaling pathway and affect bone reconstruction (95). Both in vivo and in vitro mechanics experiments support the Wnt/β-catenin signal transduction pathway as a normal response to mechanical stimulation. Wnt/β-catenin signal transduction can increase the sensitivity of bone cells to bone formation, resulting in increased bone mass and density. Biological force can induce β-catenin in bone cells to accumulate in the cytoplasm and migrate toward the nucleus, regulating gene transcription and expression. Studies have shown that fluid stimulation can release PGE2 through the β-catenin signaling pathway (72,96). Also, previous research indicates that the protein associated with the Wnt signaling pathway in osteocyte-like cells after pulsatile fluid action are all up-regulated, including Wnt-3a, secreted frizzled-related protein 4 (sFRP-4), low density lipoprotein receptor-related protein (Lrp)-5, Lrp-6, and β-catenin. In this experiment, the expression of the Wnt classic target gene Cx43 was significantly reduced under the action of Wnt antagonists. This confirms that the Wnt signaling pathway is involved in the role of Cx43 in bone reconstruction under biomechanical stimulation (95). The Wnt/Lrp5 signaling in bone cells are closely related to bone formation (97). Lrp5/6 is currently one of the most studied cell membrane receptors that bind to the Wnt protein. Studies have shown that mechanical load can increase the gene expression of Wnt3a and Lrp5, while unloading can inhibit the Wnt/β-catenin signaling pathway (98-100). As a key factor of the Wnt/β-catenin signaling pathway, studies have shown that the expression of β-catenin and nuclear transfer are regulated by mechanical stress (97,101). Cyclin D1, lymphoid enhancer-binding factor 1 (Lef1), and Cx43 are the target genes of the Wnt/β-catenin signal pathway (99). Lef1 can form a complex with T cell-specific transcription factor (Tcf) to initiate the transcription and expression of downstream genes (102,103). The expression of Cx43 is regulated by β-catenin, and the knockout of β-catenin will lead to decreased Cx43 protein expression (104).
In terms of bone formation, the Wnt/β-catenin signaling pathway can promote the proliferation of osteoblasts by regulating the expression of LRP5 (105), induce the differentiation of bone marrow mesenchymal stem cells (BMSCs) into osteoblasts by regulating bone morphogenetic protein (BMP) (106), and promote the mineralization of extracellular matrix (ECM) by promoting the mRNA expression of the extracellular matrix phosphorylated glycoprotein (MEPE) (107). In terms of bone metabolism, this pathway can act on the osteoprotegerin (OPG)/RANKL/ receptor activator of nuclear factor κB (RANK) pathway, inhibiting bone cells from expressing the OPG gene and thereby inhibiting osteoclast differentiation (108). Dickkopf-related protein 1 (DKK-1) is a negative regulator of the Wnt pathway, which can inhibit osteoblast differentiation and promote osteoclast differentiation and maturation during bone metabolism (109). The Wnt signaling pathway can not only promote the differentiation and proliferation of osteoblasts but also inhibit the development and maturation of osteoclasts, and plays an important role in the metabolic process of the skeletal system (110). Therefore, intercellular communication and signal complex association with interstitial connection channels may be interwoven functions not only by the charge and size of interstitial connection selective permeability but also by the locally recruited signal complex to determine the biological activity of Cx43. Cx43 is also directly involved in the regulation of osteoblast differentiation. It has been reported that Cx43 knockdown significantly inhibited osteoblast markers such as alkaline phosphatase, type I collagen, and fibroblast growth factor 23, resulting in a significant attenuation of osteoblast mineralization. In a bone wear model, osteolytic titanium (Ti) inhibited β-connexin expression by inducing Cx42 expression, which in turn promoted bone formation. Other studies found that overexpression of Cx43 induced alkaline phosphatase expression through elevated GJ conductance and promoted the proliferation and differentiation of UMR cells (111-113).
Conclusions and future prospects
The expression of Cx43 and its channel function are particularly important in the normal physiological activities of bone tissue cells. Despite their relatively high abundance, even in the case of non-osteoblasts, osteoblasts are probably among the least studied cells in all vertebrate biology. In osteocytes, one of the important mechanical sensing molecules is Cx43, which forms interstitial junctions and hemichannels and is involved in mechanical signal transduction between adjacent cells and between cells and the extracellular environment (107-109). Further, Cx43 has been shown to be closely associated with other common skeletal developmental disorders. Cx43 deficiency was found to further enhance estrogen deficiency-induced osteoporosis, suggesting that Cx43 protects against estrogen-induced trabecular bone loss (73). Glucocorticoids, considered to be an important etiological factor in osteoporosis, have been shown to inhibit Cx43 expression in osteoblasts through activation of the AKt/mTOR signaling pathway (114). In terms of resistance to bone aging function, it was found that young skeletally mature mice deficient in Cx43 phenocopied osteoblast apoptosis and reduced bone strength, similar to the aged mouse phenotype (115). These findings suggested that Cx43 plays an important biological function in both normal bone development and skeletal disease. Furthermore, we focused on Cx43 non-skeletal biological functions. Furthermore, we have focused on Cx43 non-skeletal biological functions, with Cx43’s role in cardiovascular disease being the most talked about most in addition to skeletal disease. Numerous studies have shown that deletion or phosphorylation of Cx43 expression in cardiovascular disease is associated with loss of cardioprotection (116,117). In the future, further investigation of the mechanisms and targets of Cx43 regulation of osteoblasts appears to be of great therapeutic value. In addition, pharmacological agents that antagonize or enhance Cx43 will also be further used and validated. For example, a series of peptides including alpha connexin carboxyl terminus 1 have been developed to target the specific activities and functions of Cx43, which will be further validated in vitro and in vivo.
In summary, osteocytes, osteoblasts, and osteoclasts all express Cx43 and form an overall network through the interaction between GJs. Cx43 plays a vital role in regulating bone cell function, signal transduction, and mechanical conduction. Clarifying the specific molecular mechanism of Cx43 in regulating bone homeostasis and responding to mechanical stimuli and signals can provide new molecular markers for certain bone diseases and novel treatment targets. Modulation of the immune microenvironment is increasingly becoming a favorable target for bone, cartilage, and soft tissue regeneration. The relationship between Cx43 and the bone microenvironment and its intrinsic mechanisms hold promise for new therapeutic opportunities to enhance bone, cartilage, and soft tissue regeneration through modulation of the immune microenvironment.
Acknowledgments
Funding: The study was supported by Shandong Taishan Scholars Project (No. tsqn202211350 to P.L.); Shandong Province Key R&D Program (Major Technological Innovation Project) (No. 2021CXGC010501); Shandong Provincial Natural Science Foundation (No. ZR2021MH071); China Postdoctoral Science Foundation (Nos. 2020M682220 and 2021T140423).
Footnote
Reporting Checklist: The authors have completed the Narrative Review reporting checklist. Available at https://aoj.amegroups.com/article/view/10.21037/aoj-23-65/rc
Peer Review File: Available at https://aoj.amegroups.com/article/view/10.21037/aoj-23-65/prf
Conflicts of Interest: All authors have completed the ICMJE uniform disclosure form (available at https://aoj.amegroups.com/article/view/10.21037/aoj-23-65/coif). The authors have no conflicts of interest to declare.
Ethical Statement: The authors are accountable for all aspects of the work in ensuring that questions related to the accuracy or integrity of any part of the work are appropriately investigated and resolved.
Open Access Statement: This is an Open Access article distributed in accordance with the Creative Commons Attribution-NonCommercial-NoDerivs 4.0 International License (CC BY-NC-ND 4.0), which permits the non-commercial replication and distribution of the article with the strict proviso that no changes or edits are made and the original work is properly cited (including links to both the formal publication through the relevant DOI and the license). See: https://creativecommons.org/licenses/by-nc-nd/4.0/.
References
- Bonewald LF. Osteocytes as dynamic multifunctional cells. Ann N Y Acad Sci 2007;1116:281-90. [Crossref] [PubMed]
- Robling AG, Bonewald LF. The Osteocyte: New Insights. Annu Rev Physiol 2020;82:485-506. [Crossref] [PubMed]
- Bonewald LF. Generation and function of osteocyte dendritic processes. J Musculoskelet Neuronal Interact 2005;5:321-4. [PubMed]
- Inaba N, Kuroshima S, Uto Y, et al. Cyclic mechanical stretch contributes to network development of osteocyte-like cells with morphological change and autophagy promotion but without preferential cell alignment in rat. Biochem Biophys Rep 2017;11:191-7. [Crossref] [PubMed]
- Carpintero-Fernandez P, Gago-Fuentes R, Wang HZ, et al. Intercellular communication via gap junction channels between chondrocytes and bone cells. Biochim Biophys Acta Biomembr 2018;1860:2499-505. [Crossref] [PubMed]
- Loiselle AE, Jiang JX, Donahue HJ. Gap junction and hemichannel functions in osteocytes. Bone 2013;54:205-12. [Crossref] [PubMed]
- Batra N, Kar R, Jiang JX. Gap junctions and hemichannels in signal transmission, function and development of bone. Biochim Biophys Acta 2012;1818:1909-18. [Crossref] [PubMed]
- Hammond MA, Berman AG, Pacheco-Costa R, et al. Removing or truncating connexin 43 in murine osteocytes alters cortical geometry, nanoscale morphology, and tissue mechanics in the tibia. Bone 2016;88:85-91. [Crossref] [PubMed]
- Vinken M. Introduction: connexins, pannexins and their channels as gatekeepers of organ physiology. Cell Mol Life Sci 2015;72:2775-8. [Crossref] [PubMed]
- Maeda S, Tsukihara T. Structure of the gap junction channel and its implications for its biological functions. Cell Mol Life Sci 2011;68:1115-29. [Crossref] [PubMed]
- Plotkin LI. Connexin 43 and bone: not just a gap junction protein. Actual Osteol 2011;7:79-90. [PubMed]
- Plotkin LI, Bellido T. Beyond gap junctions: Connexin43 and bone cell signaling. Bone 2013;52:157-66. [Crossref] [PubMed]
- Esseltine JL, Laird DW. Next-Generation Connexin and Pannexin Cell Biology. Trends Cell Biol 2016;26:944-55. [Crossref] [PubMed]
- Stains JP, Civitelli R. Gap junctions in skeletal development and function. Biochim Biophys Acta 2005;1719:69-81. [Crossref] [PubMed]
- Weinbaum S, Cowin SC, Zeng Y. A model for the excitation of osteocytes by mechanical loading-induced bone fluid shear stresses. J Biomech 1994;27:339-60. [Crossref] [PubMed]
- Stains JP, Watkins MP, Grimston SK, et al. Molecular mechanisms of osteoblast/osteocyte regulation by connexin43. Calcif Tissue Int 2014;94:55-67. [Crossref] [PubMed]
- Rodjakovic D, Salm L, Beldi G. Function of Connexin-43 in Macrophages. Int J Mol Sci 2021;22:1412. [Crossref] [PubMed]
- Talbot J, Dupuy M, Morice S, et al. Antagonistic Functions of Connexin 43 during the Development of Primary or Secondary Bone Tumors. Biomolecules 2020;10:1240. [Crossref] [PubMed]
- Wang GG, Wang Y, Wang SL, et al. Down-regulation of CX43 expression by miR-1 inhibits the proliferation and invasion of glioma cells. Transl Cancer Res 2022;11:4126-36. [Crossref] [PubMed]
- Tian Y, Riquelme MA, Tu C, et al. Osteocytic Connexin Hemichannels Modulate Oxidative Bone Microenvironment and Breast Cancer Growth. Cancers (Basel) 2021;13:6343. [Crossref] [PubMed]
- Bonacquisti EE, Nguyen J. Connexin 43 (Cx43) in cancer: Implications for therapeutic approaches via gap junctions. Cancer Lett 2019;442:439-44. [Crossref] [PubMed]
- Huang Y, Liu W, Liu Y, et al. Glycated serum albumin decreases connexin 43 phosphorylation in the corpus cavernosum. Transl Androl Urol 2022;11:1486-94. [Crossref] [PubMed]
- Yang HT, Li LL, Li SN, et al. MicroRNA-155 inhibition attenuates myocardial infarction-induced connexin 43 degradation in cardiomyocytes by reducing pro-inflammatory macrophage activation. Cardiovasc Diagn Ther 2022;12:325-39. [Crossref] [PubMed]
- Yao J, Ke J, Zhou Z, et al. Combination of HGF and IGF-1 promotes connexin 43 expression and improves ventricular arrhythmia after myocardial infarction through activating the MAPK/ERK and MAPK/p38 signaling pathways in a rat model. Cardiovasc Diagn Ther 2019;9:346-54. [Crossref] [PubMed]
- Gao J, Cheng TS, Qin A, et al. Glucocorticoid impairs cell-cell communication by autophagy-mediated degradation of connexin 43 in osteocytes. Oncotarget 2016;7:26966-78. [Crossref] [PubMed]
- Cheng B, Zhao S, Luo J, et al. Expression of functional gap junctions and regulation by fluid flow in osteocyte-like MLO-Y4 cells. J Bone Miner Res 2001;16:249-59. [Crossref] [PubMed]
- Plotkin LI, Stains JP. Connexins and pannexins in the skeleton: gap junctions, hemichannels and more. Cell Mol Life Sci 2015;72:2853-67. [Crossref] [PubMed]
- Jiang JX, Cherian PP. Hemichannels formed by connexin 43 play an important role in the release of prostaglandin E(2) by osteocytes in response to mechanical strain. Cell Commun Adhes 2003;10:259-64. [PubMed]
- Lloyd SA, Loiselle AE, Zhang Y, et al. Shifting paradigms on the role of connexin43 in the skeletal response to mechanical load. J Bone Miner Res 2014;29:275-86. [Crossref] [PubMed]
- Batra N, Burra S, Siller-Jackson AJ, et al. Mechanical stress-activated integrin α5β1 induces opening of connexin 43 hemichannels. Proc Natl Acad Sci U S A 2012;109:3359-64. [Crossref] [PubMed]
- Riquelme MA, Gu S, Hua R, et al. Mechanotransduction via the coordinated actions of integrins, PI3K signaling and Connexin hemichannels. Bone Res 2021;9:8. [Crossref] [PubMed]
- Siller-Jackson AJ, Burra S, Gu S, et al. Adaptation of connexin 43-hemichannel prostaglandin release to mechanical loading. J Biol Chem 2008;283:26374-82. [Crossref] [PubMed]
- Gluhak-Heinrich J, Gu S, Pavlin D, et al. Mechanical loading stimulates expression of connexin 43 in alveolar bone cells in the tooth movement model. Cell Commun Adhes 2006;13:115-25. [Crossref] [PubMed]
- Loiselle AE, Paul EM, Lewis GS, et al. Osteoblast and osteocyte-specific loss of Connexin43 results in delayed bone formation and healing during murine fracture healing. J Orthop Res 2013;31:147-54. [Crossref] [PubMed]
- Plotkin LI, Lezcano V, Thostenson J, et al. Connexin 43 is required for the anti-apoptotic effect of bisphosphonates on osteocytes and osteoblasts in vivo. J Bone Miner Res 2008;23:1712-21. [Crossref] [PubMed]
- Grimston SK, Brodt MD, Silva MJ, et al. Attenuated response to in vivo mechanical loading in mice with conditional osteoblast ablation of the connexin43 gene (Gja1). J Bone Miner Res 2008;23:879-86. [Crossref] [PubMed]
- Davis HM, Pacheco-Costa R, Atkinson EG, et al. Disruption of the Cx43/miR21 pathway leads to osteocyte apoptosis and increased osteoclastogenesis with aging. Aging Cell 2017;16:551-63. [Crossref] [PubMed]
- Zhao D, Liu R, Li G, et al. Connexin 43 Channels in Osteocytes Regulate Bone Responses to Mechanical Unloading. Front Physiol 2020;11:299. [Crossref] [PubMed]
- Buo AM, Stains JP. Gap junctional regulation of signal transduction in bone cells. FEBS Lett 2014;588:1315-21. [Crossref] [PubMed]
- Suswillo RF, Javaheri B, Rawlinson SC, et al. Strain uses gap junctions to reverse stimulation of osteoblast proliferation by osteocytes. Cell Biochem Funct 2017;35:56-65. [Crossref] [PubMed]
- Lloyd SA, Loiselle AE, Zhang Y, et al. Connexin 43 deficiency desensitizes bone to the effects of mechanical unloading through modulation of both arms of bone remodeling. Bone 2013;57:76-83. [Crossref] [PubMed]
- Bivi N, Pacheco-Costa R, Brun LR, et al. Absence of Cx43 selectively from osteocytes enhances responsiveness to mechanical force in mice. J Orthop Res 2013;31:1075-81. [Crossref] [PubMed]
- Grimston SK, Watkins MP, Stains JP, et al. Connexin43 modulates post-natal cortical bone modeling and mechano-responsiveness. Bonekey Rep 2013;2:446. [Crossref] [PubMed]
- Musil LS, Goodenough DA. Biochemical analysis of connexin43 intracellular transport, phosphorylation, and assembly into gap junctional plaques. J Cell Biol 1991;115:1357-74. [Crossref] [PubMed]
- Alford AI, Jacobs CR, Donahue HJ. Oscillating fluid flow regulates gap junction communication in osteocytic MLO-Y4 cells by an ERK1/2 MAP kinase-dependent mechanism. Bone 2003;33:64-70. [Crossref] [PubMed]
- Jørgensen NR, Teilmann SC, Henriksen Z, et al. Activation of L-type calcium channels is required for gap junction-mediated intercellular calcium signaling in osteoblastic cells. J Biol Chem 2003;278:4082-6. [Crossref] [PubMed]
- Ishihara Y, Sugawara Y, Kamioka H, et al. In situ imaging of the autonomous intracellular Ca(2+) oscillations of osteoblasts and osteocytes in bone. Bone 2012;50:842-52. [Crossref] [PubMed]
- Ishihara Y, Sugawara Y, Kamioka H, et al. Ex vivo real-time observation of Ca(2+) signaling in living bone in response to shear stress applied on the bone surface. Bone 2013;53:204-15. [Crossref] [PubMed]
- Saunders MM, You J, Trosko JE, et al. Gap junctions and fluid flow response in MC3T3-E1 cells. Am J Physiol Cell Physiol 2001;281:C1917-25. [Crossref] [PubMed]
- Zhou JZ, Riquelme MA, Gu S, et al. Osteocytic connexin hemichannels suppress breast cancer growth and bone metastasis. Oncogene 2016;35:5597-607. [Crossref] [PubMed]
- Cherian PP, Siller-Jackson AJ, Gu S, et al. Mechanical strain opens connexin 43 hemichannels in osteocytes: a novel mechanism for the release of prostaglandin. Mol Biol Cell 2005;16:3100-6. [Crossref] [PubMed]
- Gu G, Nars M, Hentunen TA, et al. Isolated primary osteocytes express functional gap junctions in vitro. Cell Tissue Res 2006;323:263-71. [Crossref] [PubMed]
- Klein-Nulend J, Bakker AD, Bacabac RG, et al. Mechanosensation and transduction in osteocytes. Bone 2013;54:182-90. [Crossref] [PubMed]
- Jiang JX, Siller-Jackson AJ, Burra S. Roles of gap junctions and hemichannels in bone cell functions and in signal transmission of mechanical stress. Front Biosci 2007;12:1450-62. [Crossref] [PubMed]
- Genetos DC, Kephart CJ, Zhang Y, et al. Oscillating fluid flow activation of gap junction hemichannels induces ATP release from MLO-Y4 osteocytes. J Cell Physiol 2007;212:207-14. [Crossref] [PubMed]
- Chan ME, Lu XL, Huo B, et al. A Trabecular Bone Explant Model of Osteocyte-Osteoblast Co-Culture for Bone Mechanobiology. Cell Mol Bioeng 2009;2:405-15. [Crossref] [PubMed]
- O'Brien CA, Nakashima T, Takayanagi H. Osteocyte control of osteoclastogenesis. Bone 2013;54:258-63. [Crossref] [PubMed]
- Schaffler MB, Cheung WY, Majeska R, et al. Osteocytes: master orchestrators of bone. Calcif Tissue Int 2014;94:5-24. [Crossref] [PubMed]
- Riquelme MA, Cardenas ER, Xu H, et al. The Role of Connexin Channels in the Response of Mechanical Loading and Unloading of Bone. Int J Mol Sci 2020;21:1146. [Crossref] [PubMed]
- Burger EH, Klein-Nulend J. Mechanotransduction in bone--role of the lacuno-canalicular network. FASEB J 1999;13:S101-12. [Crossref] [PubMed]
- Loiselle AE, Lloyd SA, Paul EM, et al. Inhibition of GSK-3β rescues the impairments in bone formation and mechanical properties associated with fracture healing in osteoblast selective connexin 43 deficient mice. PLoS One 2013;8:e81399. [Crossref] [PubMed]
- Donahue HJ. Gap junctions and biophysical regulation of bone cell differentiation. Bone 2000;26:417-22. [Crossref] [PubMed]
- Mullender M, El Haj AJ, Yang Y, et al. Mechanotransduction of bone cells in vitro: mechanobiology of bone tissue. Med Biol Eng Comput 2004;42:14-21. [Crossref] [PubMed]
- Klein-Nulend J, van der Plas A, Semeins CM, et al. Sensitivity of osteocytes to biomechanical stress in vitro. FASEB J 1995;9:441-5. [Crossref] [PubMed]
- Reilly GC, Haut TR, Yellowley CE, et al. Fluid flow induced PGE2 release by bone cells is reduced by glycocalyx degradation whereas calcium signals are not. Biorheology 2003;40:591-603. [PubMed]
- Bonewald LF. The amazing osteocyte. J Bone Miner Res 2011;26:229-38. [Crossref] [PubMed]
- Vatsa A, Smit TH, Klein-Nulend J. Extracellular NO signalling from a mechanically stimulated osteocyte. J Biomech 2007;40:S89-95. [Crossref] [PubMed]
- Mullender MG, Dijcks SJ, Bacabac RG, et al. Release of nitric oxide, but not prostaglandin E2, by bone cells depends on fluid flow frequency. J Orthop Res 2006;24:1170-7. [Crossref] [PubMed]
- Joiner DM, Tayim RJ, McElderry JD, et al. Aged male rats regenerate cortical bone with reduced osteocyte density and reduced secretion of nitric oxide after mechanical stimulation. Calcif Tissue Int 2014;94:484-94. [Crossref] [PubMed]
- Turner CH, Forwood MR, Otter MW. Mechanotransduction in bone: do bone cells act as sensors of fluid flow? FASEB J 1994;8:875-8. [Crossref] [PubMed]
- Zhang K, Barragan-Adjemian C, Ye L, et al. E11/gp38 selective expression in osteocytes: regulation by mechanical strain and role in dendrite elongation. Mol Cell Biol 2006;26:4539-52. [Crossref] [PubMed]
- Kitase Y, Barragan L, Qing H, et al. Mechanical induction of PGE2 in osteocytes blocks glucocorticoid-induced apoptosis through both the β-catenin and PKA pathways. J Bone Miner Res 2010;25:2657-68. [Crossref] [PubMed]
- Ma L, Hua R, Tian Y, et al. Connexin 43 hemichannels protect bone loss during estrogen deficiency. Bone Res 2019;7:11. [Crossref] [PubMed]
- Cheng B, Kato Y, Zhao S, et al. PGE(2) is essential for gap junction-mediated intercellular communication between osteocyte-like MLO-Y4 cells in response to mechanical strain. Endocrinology 2001;142:3464-73. [Crossref] [PubMed]
- Li X, Liu C, Li P, et al. Connexin 43 is a potential regulator in fluid shear stress-induced signal transduction in osteocytes. J Orthop Res 2013;31:1959-65. [Crossref] [PubMed]
- Xu LH, Shao H, Ma YV, et al. OCY454 Osteocytes as an in Vitro Cell Model for Bone Remodeling Under Mechanical Loading. J Orthop Res 2019;37:1681-9. [Crossref] [PubMed]
- Thi MM, Kojima T, Cowin SC, et al. Fluid shear stress remodels expression and function of junctional proteins in cultured bone cells. Am J Physiol Cell Physiol 2003;284:C389-403. [Crossref] [PubMed]
- Yan Z, Wang P, Wu J, et al. Fluid shear stress improves morphology, cytoskeleton architecture, viability, and regulates cytokine expression in a time-dependent manner in MLO-Y4 cells. Cell Biol Int 2018;42:1410-22. [Crossref] [PubMed]
- Xu H, Liu R, Ning D, et al. Biological responses of osteocytic connexin 43 hemichannels to simulated microgravity. J Orthop Res 2017;35:1195-202. [Crossref] [PubMed]
- Matsuda J, Kurata K, Fukunaga T, et al. Bone marrow cell differentiation regulated by gel-embedded osteocyte under multi-dimensional gravity. Journal of Biomechanics 2006;39:S469-S.
- Lloyd SA, Lewis GS, Zhang Y, et al. Connexin 43 deficiency attenuates loss of trabecular bone and prevents suppression of cortical bone formation during unloading. J Bone Miner Res 2012;27:2359-72. [Crossref] [PubMed]
- Lloyd SA, Loiselle AE, Zhang Y, et al. Evidence for the role of connexin 43-mediated intercellular communication in the process of intracortical bone resorption via osteocytic osteolysis. BMC Musculoskelet Disord 2014;15:122. [Crossref] [PubMed]
- Grimston SK, Goldberg DB, Watkins M, et al. Connexin43 deficiency reduces the sensitivity of cortical bone to the effects of muscle paralysis. J Bone Miner Res 2011;26:2151-60. [Crossref] [PubMed]
- Genetos DC, Geist DJ, Liu D, et al. Fluid shear-induced ATP secretion mediates prostaglandin release in MC3T3-E1 osteoblasts. J Bone Miner Res 2005;20:41-9. [PubMed]
- Li P, Bian X, Liu C, et al. STIM1 and TRPV4 regulate fluid flow-induced calcium oscillation at early and late stages of osteoclast differentiation. Cell Calcium 2018;71:45-52. [Crossref] [PubMed]
- Mancini L, Moradi-Bidhendi N, Becherini L, et al. The biphasic effects of nitric oxide in primary rat osteoblasts are cGMP dependent. Biochem Biophys Res Commun 2000;274:477-81. [Crossref] [PubMed]
- Morrell AE, Brown GN, Robinson ST, et al. Mechanically induced Ca(2+) oscillations in osteocytes release extracellular vesicles and enhance bone formation. Bone Res 2018;6:6. [Crossref] [PubMed]
- Zhang J, Riquelme MA, Hua R, et al. Connexin 43 hemichannels regulate mitochondrial ATP generation, mobilization, and mitochondrial homeostasis against oxidative stress. Elife 2022;11:e82206. [Crossref] [PubMed]
- Li J, Liu D, Ke HZ, et al. The P2X7 nucleotide receptor mediates skeletal mechanotransduction. J Biol Chem 2005;280:42952-9. [Crossref] [PubMed]
- Suadicani SO, Brosnan CF, Scemes E. P2X7 receptors mediate ATP release and amplification of astrocytic intercellular Ca2+ signaling. J Neurosci 2006;26:1378-85. [Crossref] [PubMed]
- You L, Temiyasathit S, Lee P, et al. Osteocytes as mechanosensors in the inhibition of bone resorption due to mechanical loading. Bone 2008;42:172-9. [Crossref] [PubMed]
- Gago-Fuentes R, Bechberger JF, Varela-Eirin M, et al. The C-terminal domain of connexin43 modulates cartilage structure via chondrocyte phenotypic changes. Oncotarget 2016;7:73055-67. [Crossref] [PubMed]
- Jeon YM, Kook SH, Rho SJ, et al. Fibroblast growth factor-7 facilitates osteogenic differentiation of embryonic stem cells through the activation of ERK/Runx2 signaling. Mol Cell Biochem 2013;382:37-45. [Crossref] [PubMed]
- McCutcheon S, Majeska RJ, Spray DC, et al. Apoptotic Osteocytes Induce RANKL Production in Bystanders via Purinergic Signaling and Activation of Pannexin Channels. J Bone Miner Res 2020;35:966-77. [Crossref] [PubMed]
- Jansen JH, Eijken M, Jahr H, et al. Stretch-induced inhibition of Wnt/beta-catenin signaling in mineralizing osteoblasts. J Orthop Res 2010;28:390-6. [Crossref] [PubMed]
- Kamel MA, Picconi JL, Lara-Castillo N, et al. Activation of β-catenin signaling in MLO-Y4 osteocytic cells versus 2T3 osteoblastic cells by fluid flow shear stress and PGE2: Implications for the study of mechanosensation in bone. Bone 2010;47:872-81. [Crossref] [PubMed]
- Monroe DG, McGee-Lawrence ME, Oursler MJ, et al. Update on Wnt signaling in bone cell biology and bone disease. Gene 2012;492:1-18. [Crossref] [PubMed]
- Robling AG, Niziolek PJ, Baldridge LA, et al. Mechanical stimulation of bone in vivo reduces osteocyte expression of Sost/sclerostin. J Biol Chem 2008;283:5866-75. [Crossref] [PubMed]
- Robinson JA, Chatterjee-Kishore M, Yaworsky PJ, et al. Wnt/beta-catenin signaling is a normal physiological response to mechanical loading in bone. J Biol Chem 2006;281:31720-8. [Crossref] [PubMed]
- Niziolek PJ, Warman ML, Robling AG. Mechanotransduction in bone tissue: The A214V and G171V mutations in Lrp5 enhance load-induced osteogenesis in a surface-selective manner. Bone 2012;51:459-65. [Crossref] [PubMed]
- Yang Z, Bidwell JP, Young SR, et al. Nmp4/CIZ inhibits mechanically induced beta-catenin signaling activity in osteoblasts. J Cell Physiol 2010;223:435-41. [Crossref] [PubMed]
- Staal FJ, Meeldijk J, Moerer P, et al. Wnt signaling is required for thymocyte development and activates Tcf-1 mediated transcription. Eur J Immunol 2001;31:285-93. [Crossref] [PubMed]
- Sharma M, Jamieson C, Johnson M, et al. Specific Armadillo repeat sequences facilitate β-catenin nuclear transport in live cells via direct binding to nucleoporins Nup62, Nup153, and RanBP2/Nup358. J Biol Chem 2016;291:4342. [Crossref] [PubMed]
- Xia X, Batra N, Shi Q, et al. Prostaglandin promotion of osteocyte gap junction function through transcriptional regulation of connexin 43 by glycogen synthase kinase 3/beta-catenin signaling. Mol Cell Biol 2010;30:206-19. [Crossref] [PubMed]
- Haÿ E, Laplantine E, Geoffroy V, et al. N-cadherin interacts with axin and LRP5 to negatively regulate Wnt/beta-catenin signaling, osteoblast function, and bone formation. Mol Cell Biol 2009;29:953-64. [Crossref] [PubMed]
- Naito H, Dohi Y, Zimmermann WH, et al. The effect of mesenchymal stem cell osteoblastic differentiation on the mechanical properties of engineered bone-like tissue. Tissue Eng Part A 2011;17:2321-9. [Crossref] [PubMed]
- Sun Y, Jiang Y, Liu Q, et al. Biomimetic engineering of nanofibrous gelatin scaffolds with noncollagenous proteins for enhanced bone regeneration. Tissue Eng Part A 2013;19:1754-63. [Crossref] [PubMed]
- Chen B, Li XD, Liu DX, et al. Canonical Wnt signaling is required for Panax notoginseng saponin-mediated attenuation of the RANKL/OPG ratio in bone marrow stromal cells during osteogenic differentiation. Phytomedicine 2012;19:1029-34. [Crossref] [PubMed]
- Tao K, Xiao D, Weng J, et al. Berberine promotes bone marrow-derived mesenchymal stem cells osteogenic differentiation via canonical Wnt/β-catenin signaling pathway. Toxicol Lett 2016;240:68-80. [Crossref] [PubMed]
- Song L, Liu M, Ono N, et al. Loss of wnt/β-catenin signaling causes cell fate shift of preosteoblasts from osteoblasts to adipocytes. J Bone Miner Res 2012;27:2344-58. [Crossref] [PubMed]
- Hua R, Gu S, Jiang JX. Connexin 43 Hemichannels Regulate Osteoblast to Osteocyte Differentiation. Front Cell Dev Biol 2022;10:892229. [Crossref] [PubMed]
- Chai H, Huang Q, Jiao Z, et al. Osteocytes Exposed to Titanium Particles Inhibit Osteoblastic Cell Differentiation via Connexin 43. Int J Mol Sci 2023;24:10864. [Crossref] [PubMed]
- Gramsch B, Gabriel HD, Wiemann M, et al. Enhancement of connexin 43 expression increases proliferation and differentiation of an osteoblast-like cell line. Exp Cell Res 2001;264:397-407. [Crossref] [PubMed]
- Shen C, Kim MR, Noh JM, et al. Glucocorticoid Suppresses Connexin 43 Expression by Inhibiting the Akt/mTOR Signaling Pathway in Osteoblasts. Calcif Tissue Int 2016;99:88-97. [Crossref] [PubMed]
- Davis HM, Aref MW, Aguilar-Perez A, et al. Cx43 overexpression in osteocytes prevents osteocyte apoptosis and preserves cortical bone quality in aging mice. JBMR Plus 2018;2:206-16. [Crossref] [PubMed]
- Boengler K, Schulz R. Connexin 43 and Mitochondria in Cardiovascular Health and Disease. Adv Exp Med Biol 2017;982:227-46. [Crossref] [PubMed]
- Sedovy MW, Leng X, Leaf MR, et al. Connexin 43 across the Vasculature: Gap Junctions and Beyond. J Vasc Res 2023;60:101-13. [Crossref] [PubMed]
(English Language Editor: A. Kassem)
Cite this article as: Ma L, Wang W, Xu G, Li H, Liu F, Shao H, Zhang X, Ma Y, Li G, Li H, Gao S, Ling P. Connexin 43 in the function and homeostasis of osteocytes: a narrative review. Ann Joint 2024;9:10.