Wear and performance of a tripolar total hip replacement
Introduction
Dislocation or fear of dislocation are common and unwelcome thoughts for both patients and surgeons involved with total hip replacements (THR) (1-5). Tripolar total hip replacement was created to obtain a stable and natural range of motion (ROM). Tripolar prostheses combine the validated concepts of a two-piece cementless metal/polyethylene acetabular component and a conventional bipolar prosthesis. In tripolar hip replacement, a conventional a two-piece acetabular component is used but the polyethylene liner inner dimension is 41–54 mm rather than 22–36 mm. A standard bipolar prosthesis is then matched to this resurfacing-type acetabular component (6,7) (Figures 1,2). With the 10-fold improvement in wear resistance of highly cross-linked polyethylene, it is appropriate to reconsider the choice of a tripolar prosthesis when additional stability is needed (2,8). Dual mobility and tripolar hip designs have been described as providing less chance of dislocation, greater ROM, and more normal function compared to conventional THR. In contrast, they have also been described as unnecessarily more complicated, not any better, more productive of wear debris, and for the dual mobility prostheses, prone to intraprosthetic dislocation (9-12). Tripolar hips have not been used commonly. Tripolar hip constructs convert a conventional 28 mm THR into an average 47 mm diameter THR.
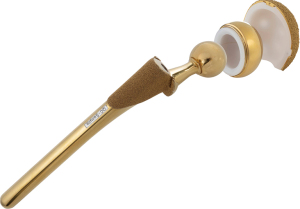
Three hypotheses form the basis for the mobile-bearing hip concept: (I) the introduction of an additional bearing surface reduces trunnion stress and, therefore, corrosion, (II) the larger diameter articulation allows for greater ROM before dislocation occurs (increased jump distance), (III) volumetric wear is reduced by sharing the motion between two articulations rather than one.
The first successful use of a tripolar prosthesis was in 1972, when a hip resurfacing patient experienced a femoral failure, but the polyethylene acetabular component remained unworn and well-fixed (13,14). A revision procedure matching a bipolar prosthesis to the acetabular component was performed (7). It was quickly found that using a bipolar prosthesis with a resurfacing acetabular prosthesis created a hip replacement with a ROM that nearly matched that of the contralateral normal hip. Primary THR using this implant combination was reported in 1979. Its initial use was in high-demand physically active patients (15).In a tripolar prosthesis, the articulation between the bipolar and the acetabular liner provides an additional area of low-friction motion. This assembly provides freedom of movement and stability. Also, the femoral head on the stem can be limited to 28 mm, thereby reducing trunnion corrosion (8). For a bipolar prosthesis, it is difficult to measure the amount of wear of the interior polyethylene articulation, as it is hidden by the metal exterior (16-18). There is no literature reporting the wear and performance of tripolar prostheses except for constrained implants, which are completely different and subject to extremely high wear.
In the 1980s, it was concluded that the minimal safe thickness of polyethylene was 8 mm (19). Because polyethylene thickness of 3–4 mm is needed for successful polyethylene hip resurfacing and tripolar component use, polyethylene hip resurfacing and tripolar hip replacement all but disappeared. There is now favorable clinical experience and increasing favorable wear simulator experience with highly cross-linked polyethylene of 3–4 mm thickness (20-23) (Table 1).
Table 1
Study | Diameter/thickness, mm | Linear, mm/year | Volumetric, mm3/year | Gravimetric, mg/mc |
---|---|---|---|---|
Retrievals (this study) | 48/4 | 0.009 | 5.6 | 5.4 |
Simulator (this study) | 52.5/3.1 | 0.0038 | 7.1 | 6.64 |
Warburton (2018)† | 24/1.5 | 0.028 | 0.28 | 0.99 |
Pritchett (2016) | 51/4 | 0.005 | 9.4 | 8.8 |
Johnson (2014) | 36/1.9 | 0.015 | 5.0 | 2.4 |
Bragdon (2001) | 46/3 | 0.014 | †† | †† |
Kelly (2010) | 44/3.8 | †† | †† | 2.3 |
Shen (2017) | 36/3 | †† | 6.3 | 5.0 |
†, Warburton Ref. (24); ††, not reported.
The purpose of this study was to evaluate wear, oxidation, particle generation, mechanical performance, and function of a tripolar hip. The present study addressed the following questions: (I) what is the wear of the tripolar prosthesis? (II) what parts of the prosthesis contribute to wear and in what proportions? (III) do component parts stay together and function as designed? (IV) how does the wear of a tripolar prosthesis compare to the wear of both conventional and dual mobility hip prostheses? I present the following article in accordance with the TREND reporting checklist (available at https://aoj.amegroups.com/article/view/10.21037/aoj-21-7/rc).
Methods
This study evaluated 24 retrieved tripolar prostheses (23 patients) implanted between February 1999 and February 2016. The study was conducted in accordance with the Declaration of Helsinki (as revised in 2013). The study was approved by Institutional Review Board of Swedish Medical Center (study number S1905-11) and informed consent was obtained from all the participants.
Explanted components were retrieved and cleaned using institutional procedures and stored in a subzero freezer to minimize ex vivo oxidative changes. The implants were retrieved from 12 women and 11 men with an average age at surgery of 49 years (range, 36–70 years) and mean weight of 78 kg (range, 54–110 kg). The diagnosis requiring THR was osteoarthritis (15), revision for recurrent dislocation (6),femoral neck fracture (2), and avascular necrosis (1). Implants were retrieved postmortem from 15 patients and during revision procedures in eight patients performed for infection (5), periprosthetic fracture (3). The time from the initial surgery to implant retrieval ranged from 5–22 years (mean, 14 years). The retrieval laboratory did not receive any failed tripolar implants and there no reports of mechanical failure of this bipolar or tripolar prosthesis. Clinical and radiographic data were available for all patients prior to implant retrieval. All patients were evaluated for osteolysis and implant loosening. The UCLA activity score and hip flexion were recorded for each patient premortem. Radiographs were taken in the neutral position as well as at the limits of abduction to determine the movement of the component parts (Figure 3A,3B).
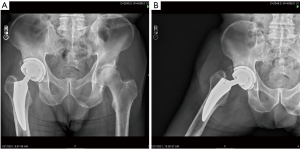
The self-aligning bipolar prosthesis 510K 848889 and the New Jersey acetabular component 510K 848888 were used (SynovoPro, Fullerton, CA, USA). When used together they have been called the Trifecta Tripolar Total Hip Replacement. The components were: (I) femoral head (II) polyethylene bearing insert that snap fits on the femoral head, (III) ceramic-coated metal head that press fits on the bipolar polyethylene bearing insert, and (IV) fixed 2-piece acetabular component consisting of a titanium metal backing and a highly cross-linked polyethylene insert. A 28 mm modular ceramic-coated femoral head was used in each case. The acetabular liners were from 41–54 mm inner diameter. The components were placed without cement (Figure 1). The bipolar prosthesis self-aligns with the load vector and positive eccentricity is built into the bearing insert. The outside spherical surface of the bearing is made eccentric by adding polyethylene at the periphery using an engineering calculation (25,26). Such eccentricity produces a small force couple when the cup is not aligned with a joint vector, which provides a self-correction to alignment (12). Most bipolar implants place additional polyethylene at the dome, and this creates negative eccentricity (Figure 4A,4B). The bipolar polyethylene has two slits and is snap fit on the femoral head and secured with a retaining ring. Six keyways allow release of the bipolar polyethylene using an extraction ring from the covering TiN-coated shell.
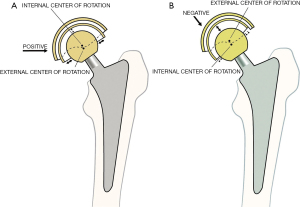
The acetabular bearings are seated into a TiAl6V4 shell. The shells are hemispherical with inferiorly placed anterior and posterior extensions and an anatomic inferior cut out (Figure 1). The acetabular shell has three keyways that resist spin out and flexible locking tabs that engage a groove in the acetabular shell. The acetabular and bipolar polyethylene are from 3.23–3.68 mm in minimum thickness. The acetabular shells are 2 mm thick and the coating for cementless fixation adds another 1 mm (13,21,22). The polyethylene bearings are GUR 1020 cross-linked at 7.5 Mrad. They are treated with remelting at 155 °F to limit free radicals and sterilized with ethylene oxide. The TiN ceramic surface layer coating on the bipolar femoral head was 8 µm and it was deposited using a physical vapor deposition process (Ion Bond, LLC, Rockaway, NJ, USA). The radial clearance was a minimum of 300 µm and the surface roughness of the femoral component was <3 µm. The median diameter of the outer head was 47 mm (range, 41–54 mm). The retrieved polyethylene liners and bipolar polyethylene inserts were analyzed for wear using gravimetric, geometric, 3D light evaluation, morphologic appearance, and machining marks. The morphologic (visual) analysis was performed with the unaided eye and with stereomicroscopic magnification of 20×. The dimensions of both the bipolar polyethylene insert and acetabular liner insert were compared to the manufacturing blueprints and measurements of unused implants. The measured change in the dimensions divided by the number of years of use was used to calculate the wear rate. This retrieval study was conducted using ASTM F561-97 (27) and ISO 12891 (28).
Oxidation index testing
The polyethylene components were visually inspected looking for white banding, delamination, and cracks. Fourier transform infrared (FTIR) spectroscopy was performed and the oxidation index was assigned using ASTM F2102 (29).
Damage assessment
During the morphologic examination, the rim was given particular attention for seven patterns of damage: (I) abrasion, (II) burnishing, (III) scratching, (IV) deformation, (V) embedded particles, (VI) pitting, and (VII) delamination. A damage score following the modified Hood method was assigned (30). The scores for each type of polyethylene damage ranged from 0–3 and the acetabular and bipolar polyethylene were divided into seven sections.
Volumetric wear
Volumetric wear was measured with a digital Coordinate Measuring Machine (CMM) (Mitutoyo America Corporation, Aurora, IL, USA). The CMM measurements were compared to scans of size-matched never used implants provided by the manufacturer, who also provided blueprints. A best-fit comparison analysis was performed. The details of the CMM data collection have described elsewhere (31).
3D optical scan
Recently, 3D optical scanners have been used to measure wear in total knee replacements, dual mobility prostheses, and hip resurfacing polyethylene liners. This technology is much faster and less costly than CMM measurements. It also is nondestructive and has been validated as accurate to within 0.18–0.46% compared to CMM. This technique has been described elsewhere (25,32,33). The ZfxTM system (Zimmer/Biomet, Palm Beach Gardens, FL, USA) was used. This system is used commonly in dental applications.
Gravimetric wear analysis
The retrieval analysis also included determining the weight of the bipolar and acetabular polyethylene implants. This weight was compared to the known weight for the dimension as available from the manufacturer. Gravimetric analyses are used most often in wear simulator studies but can also provide useful information in retrieval studies. The known weight from the manufacturer, including soak controls using calf serum, were used to simulate the lubrication of implants in the body. The typical weight of an acetabular polyethylene liner was approximately 6.65 grams. The bipolar polyethylene liners weighed from 10–24 grams depending on dimension. Six unused 52.5/3.1 mm polyethylene liners were also wear-simulator tested for 5 million cycles per ISO 14242-1 (34).
Mechanical testing
The forces necessary to separate the bipolar and acetabular prostheses were measured using ASTM F1820. Tests for push-out, torque-out and lever-out were performed. The acceptance criteria for these are 200 N, 400 N* cm, and 3,000 N* cm, respectively. Fatigue strength of both the acetabular shell and bipolar was tested according to ASTM F2068 with the acceptance criteria of 5,240 N. Separation tests for both the acetabular liner from the shell and the bipolar head from the femoral head were performed and the acceptance level for this is 490 N. The jump distance was determined. The Semlitsch endurance test for the assembled tripolar was run to 10 M cycles.
ROM testing
ROM testing was performed premortem.
Statistical analysis
All statistical analyses were performed using the statistical software SPSS v. 19 (SPSS, Chicago, IL, USA). Descriptive statistics were displayed as mean, range, and standard deviations. Results are reported using a best fit analysis when appropriate. Wear rates and measurements were performed by two reviewers and intra-class correlation was used to assess inter-observer reliability. Standard statistical tests including Student’s t-test were used. A P value of <0.05 was considered significant.
Results
The results of wear testing are shown in Table 2. None of the retrieved implants had a mechanical failure. The median pre-mortem hip flexion was 145°±20°. The mean UCLA activity score was 7.9. No implants had radiographic or clinical signs of osteolysis or loosening. Twenty-two of 24 implants (92%) showed wear on the rim of the bipolar polyethylene visible with the unaided eye, 11 of 24 (46%) showed limited delamination of the rim, and 11 of 24 showed rim abrasions. The average abrasion depth was 0.14 mm/yr. (range, 0.02–0.34 mm/yr). The mean wear rate for the 6 wear-simulator tested 52.5/3.1 mm samples was 6.64±1.03 mg/mc. Wear was not related to patient activity score, age, or diagnosis. Most wear particles were of submicron size with an area smaller than 0.5 µm2. The number of particles in the capsular tissues was 0.75 million/gram (range, 0.1–1.9 million/gram). Electron microscopic examination of the periarticular tissues showed rare macrophages and limited inflammation. There were no signs of an adverse wear reaction.
Table 2
Wear tested | Component part | Results |
---|---|---|
Median volumetric wear, mm3/year | Bipolar bearing inner dome | 7.1±4.2 |
Bipolar bearing convex side | 11.3±7.3 | |
Acetabular polyethylene liner | 5.6±1.7 | |
Acetabular liner + bipolar polyethylene | 24.0±7.6 | |
Median gravimetric wear, mg/mc | Acetabular polyethylene liner | 5.5±1.03 |
Total gravimetric wear, mm3/year | Bipolar bearing | 13.7±10 |
Median linear wear, mm/year | Bipolar bearing | 0.19±0.11 |
Acetabular polyethylene liner | 0.009±0.008 | |
Mean TiN wear, µm | Pole of the bipolar head | 1.85 |
Mean total wear, µm | Pole of the femoral head | 1.5 |
Mean oxidation index | Acetabular polyethylene | 0.2 (max, 1.0) |
Bipolar polyethylene | 0.4 (max, 1.5) | |
Mean damage score | Acetabular polyethylene | 4.7 (max, 16) |
Bipolar polyethylene | 7.6 (max, 17) |
The results of mechanical testing are shown in Table 3. Both the bipolar inner articulation and outer articulation with the acetabular bearing continued to show the expected movement on both visual and radiographic examination in all cases (Figure 3A,3B). The endurance test to 10 M cycles showed no failures which exceeds 2,803 N of load. The bipolar shell remained in neutral or slight valgus orientation on all radiographs. There were no signs of white banding or impending failure of any polyethylene. The original machine marks were present on all retrievals.
Table 3
Mechanical testing | Results |
---|---|
Mean jump distance | 16±2 mm |
Bipolar lever-out force | 3,667±291 N* cm |
Bipolar push-out forces | 1,004±41 N pre-fatigue, 6,160±552 N post-fatigue |
Acetabular torque force | 1,341 N* cm |
Bipolar torque force | 1,497 N* cm |
Fatigue strength of the acetabular and bipolar shells | 5,340 N at 10 mc cycles |
Acetabular liner separation force | 890±93.6 N |
Bipolar separation force | 2,180±189 N |
Mean abduction-adduction arc of motion before engagement (impingement) of the femoral neck on the bipolar | 119°±9° |
Combined internal and external rotation motion | 240°±40° |
Combined flexion and extension | 145°±30° |
Combined abduction and adduction | 145°±30° |
Flexion in 45° of internal rotation before impingement | 92°±16° |
Discussion
Both the acetabular and bipolar systems in this study have been used effectively since 1988 (25). The retrieved tripolar prostheses showed low wear and oxidation as determined by gravimetric, geometric (CMM), 3D optical analysis, and visual examination. Also, the mechanical function of the implants remained intact and faithful to the design. These retrieval results are consistent with published positive clinical outcomes of this tripolar prosthesis (13). The median combined volumetric wear was 24 mm3/yr., which is below the osteolytic volumetric wear threshold of 40 mm3/yr. It is also less than the 38 mm3/yr. of a Charnley prosthesis and 38–54 mm3/yr. of a dual mobility prosthesis (35,36). In this tripolar prosthesis, ⅔ of the wear was from the bipolar polyethylene and ⅓ was from the acetabular liner. In a dual mobility, 54% of the wear comes from the convex surface of the polyethylene and 46% from the concave inner surface. The damage scores on the retrieved acetabular liners and bipolar polyethylene showed limited damage (16-18,37,38). Linear wear can be measured directly from CMM or 3D optical analysis or mathematically derived from volumetric wear and vice versa. Therefore, it is possible to compare retrieval and wear simulator studies to radiographic linear wear studies.
The low wear in this study is consistent with the results of other studies of highly cross-linked polyethylene. Highly cross-linked polyethylene has 10× less wear than conventional polyethylene (2,8,39,40). This allows for a reduction in thickness and an increase in diameter of liners without a wear penalty. Large diameter liners create a benefit in ROM and stability (13,35,41). Comparison to other studies is possible (Table 1). All of these wear studies suggest more than a lifetime of use can be expected. The wear of TiN was very low and consistent with prior studies (12).
The wear of the tripolar implant in the present study was much less than a bipolar prosthesis. It is not surprising that adding a low-friction acetabular surface reduces overall wear compared to the higher friction of the bipolar head articulating with a native acetabulum (16-18,37,38). The oxidation index of this highly cross-linked polyethylene was extremely low and consistent with the lack of mechanical failures seen with this implant. Factors contributing to the low wear seen in this study include: (I) the use of highly cross-linked rather than conventional polyethylene, (II) the positive eccentricity of the bipolar design, (III) reduced wear from the TiN ceramic coating, (IV) ethylene oxide sterilization rather than gamma irradiation, and (V) the unconstrained design (2,8,42). Over time, many bipolar prostheses have most, if not all, motion occurring between the bipolar polyethylene bearing and femoral head rather than shared between the femoral head and acetabular surface. This low friction tripolar system-maintained motion at both articulations (Figure 3A,3B) (16-18,38). The radiographs showed that the bipolar design remained faithful to the positive eccentricity. A consistent neutral alignment was seen on each radiograph and at the retrieval. This creates favorable wear and stability.
Mechanical testing showed that very high forces were necessary to cause disassembly of the acetabular liner from its shell and the bipolar head from the femoral head. The forces necessary were several times greater than the acceptance criteria and suitable even for high demand use. This includes fatigue testing, push-out, lever-out and torque-out for both the bipolar and acetabular components. This explains the lack of component separation with both the bipolar and acetabular components during the up to 22 years of high-demand clinical use in this and other studies (5). It also explains the stability of this tripolar design. Mechanical testing showed stronger resistance to lever-out, torque-out, push-out, and liner separation compared to other implants (26,43-45). This acetabular component also has functioned well in clinical, wear, and deformation studies (20,23,46). The extended ROM and increased jump distance were also consistent with the excellent clinical function seen.
There are limitations to this work. Only one tripolar prosthetic design was studied and there were a limited number of cases. However, this is the largest retrieval study for a single type of bipolar prosthesis and the only retrieval study for an unconstrained tripolar prosthesis. The follow-up interval is the longest reported. Using several methods to assess wear is also a strength of this work. 3D optical scanning is an emerging and efficient way to perform wear assessment (25,32,34).
There are concerns about the use of tripolar hip prostheses. Implant disassembly can occur with bipolar designs, but it has not been reported with this implant despite four decades of use (24). The femoral neck is designed to impinge at the limit of the arc of motion to drive the inner bipolar articulation. Severe cases of bipolar impingement and osteolysis have been found when the movement of the outer bearing is impeded by development of high friction or a vacuum between the bipolar head and the native acetabulum. Certain patient lifestyles, however, might still lead to unfavorable neck impingement and adverse wear or component separation; however, this was not seen in this study. Debris from bipolar impingement can cause an inflammatory reaction or osteolysis. An acetabular bearing surface encourages motion at both articulations.
The main use of tripolar prostheses is to treat hip instability following conventional THR. It has been a highly successful procedure for this indication (5). Tripolar prostheses have been used occasionally in high demand patients in need of a very stable hip replacement for certain adventure sports or physically demanding occupations (13). A tripolar prosthesis can also be used to treat acetabular wear after bipolar hemiarthroplasty and femoral failure after polyethylene hip resurfacing (14). A conventional THR can be adapted easily to a tripolar design simply by adding the bipolar component to the existing femoral head and changing the polyethylene acetabular liner to a larger diameter; the shell and stem remain. Tripolar implants have a median diameter of 47 mm compared with 28–36 mm for conventional THR. Tripolar prostheses are different from dual mobility prostheses. There are four concerns for dual mobility prostheses that are not concerns for tripolar prostheses: (I) intraprosthetic dislocation can occur when the femoral head disengages from the mobile polyethylene bearing; (II) the one-piece acetabular component can present fixation challenges or if a two-piece acetabular component is used, corrosion between the metal shell and liner can occur; (III) the metal acetabular component can be a source of metal sensitivity; (IV) stress shielding of the periacetabular bone supporting the thicker and stiffer press-fit metal acetabular shell can occur (47).
Tripolar prostheses are very stable and can achieve a near-normal ROM. With wear-resistant surfaces and favorable design features, a tripolar prosthesis can provide a lifetime of high-demand use. The results of this study create a compelling argument in favor of a tripolar prosthesis as a safer technology compared to dual mobility and other options for circumstances in which stability and ROM drive the need beyond a conventional hip prosthesis.
Acknowledgments
Funding: None.
Footnote
Reporting Checklist: The author has completed the TREND reporting checklist. Available at https://aoj.amegroups.com/article/view/10.21037/aoj-21-7/rc
Data Sharing Statement: Available at https://aoj.amegroups.com/article/view/10.21037/aoj-21-7/dss
Conflicts of Interest: The author has completed the ICMJE uniform disclosure form (available at https://aoj.amegroups.com/article/view/10.21037/aoj-21-7/coif). The author has no conflicts of interest to declare.
Ethical Statement: The author is accountable for all aspects of the work (if applied, including full data access, integrity of the data and the accuracy of the data analysis) in ensuring that questions related to the accuracy or integrity of any part of the work are appropriately investigated and resolved. The study was conducted in accordance with the Declaration of Helsinki (as revised in 2013). The study was approved by Institutional Review Board of Swedish Medical Center (study number S1905-11) and informed consent was obtained from all the participants.
Open Access Statement: This is an Open Access article distributed in accordance with the Creative Commons Attribution-NonCommercial-NoDerivs 4.0 International License (CC BY-NC-ND 4.0), which permits the non-commercial replication and distribution of the article with the strict proviso that no changes or edits are made and the original work is properly cited (including links to both the formal publication through the relevant DOI and the license). See: https://creativecommons.org/licenses/by-nc-nd/4.0/.
References
- Bozic KJ, Kurtz SM, Lau E, et al. The epidemiology of revision total hip arthroplasty in the United States. J Bone Joint Surg Am 2009;91:128-33. [Crossref] [PubMed]
- Bragdon CR, Jasty M, Muratoglu OK, et al. Third-body wear testing of a highly cross-linked acetabular liner: the effect of large femoral head size in the presence of particulate poly(methyl-methacrylate) debris. J Arthroplasty 2005;20:379-85. [Crossref] [PubMed]
- Brooks PJ. Dislocation following total hip replacement: causes and cures. Bone Joint J 2013;95-B:67-9. [Crossref] [PubMed]
- Chandler RW, Dorr LD, Perry J. The functional cost of dislocation following total hip arthroplasty. Clin Orthop Relat Res 1982;168-72. [Crossref] [PubMed]
- Grigoris P, Grecula MJ, Amstutz HC. Tripolar hip replacement for recurrent prosthetic dislocation. Clin Orthop Relat Res 1994;148-55. [Crossref] [PubMed]
- Bateman JE. Single-assembly total hip prosthesis--preliminary report. 1974. Clin Orthop Relat Res 1990;3-6. [PubMed]
- Giliberty RP. Hemiarthroplasty of the hip using a low-friction bipolar endoprosthesis. Clin Orthop Relat Res 1983;86-92. [Crossref] [PubMed]
- Muratoglu OK, Bragdon CR, O’Connor D, et al. Larger diameter femoral heads used in conjunction with a highly cross-linked ultra-high molecular weight polyethylene: a new concept J Arthroplasty 2001;16:24-30. [Crossref] [PubMed]
- Adam P, Farizon F, Fessy MH. Dual mobility retentive acetabular liners and wear: surface analysis of 40 retrieved polyethylene implants. Orthop Traumatol Surg Res 2014;100:85-91. [Crossref] [PubMed]
- Wegrzyn J, Malatray M, Pibarot V, et al. Is isolated mobile component exchange an option in the management of intraprosthetic dislocation of a dual mobility cup? Clin Orthop Relat Res 2020;478:279-87. [Crossref] [PubMed]
- Revell PA, Weightman B, Freeman MA, et al. The production and biology of polyethylene wear debris. Arch Orthop Trauma Surg 1978;91:167-81. [Crossref] [PubMed]
- Osman K, Panagiotidou AP, Khan M, Blunn G, Haddad FS. Corrosion at the head-neck interface of current designs of modular femoral components: essential questions and answers relating to corrosion in modular head-neck junctions. Bone Joint J 2016;98-B:579-84. [Crossref] [PubMed]
- Pritchett JW. Adventure sports and sexual freedom hip replacement: The tripolar hip. Eur J Orthop Surg Traumatol 2018;28:37-43. [Crossref] [PubMed]
- Scheerlinck T, Casteleyn PP. "Tripolar" hip arthroplasty for failed hip resurfacing: nineteen years follow-up. Acta Orthop Belg 2001;67:407-11. [PubMed]
- Levy RN. Combined total hip-replacement. Clin Orthop Relat Res 1979;287.
- Hara K, Kaku N, Tsumora H, et al. Analysis of wear and oxidation on retrieved bipolar polyethylene liner. J Orthop Sci 2008;13:366-70. [Crossref] [PubMed]
- Hess MD, Baker EA, Salisbury MR, et al. Effect of component design in retrieved bipolar hip hemiarthroplasty systems. Orthopedics 2013;36:e1180. [Crossref] [PubMed]
- Kusaba A, Kuroki Y. Wear of bipolar hip prostheses. J Arthroplasty 1998;13:668-73. [Crossref] [PubMed]
- Bartel DL, Bicknell VL, Wright TM. The effect of conformity, thickness, and material on stresses in ultra-high molecular weight components for total joint replacement. J Bone Joint Surg Am 1986;68:1041-51. [Crossref] [PubMed]
- Pritchett J. Very Large Diameter Polymer Acetabular Liners Show Promising Wear Simulator Results. J Long Term Eff Med Implants 2016;26:311-9. [Crossref] [PubMed]
- Pritchett JW. Curved-stem hip resurfacing: Minimum 20-year follow-up. Clin Orthop Relat Res 2008;466:1177-85. [Crossref] [PubMed]
- Buechel FF Sr, Buechel FF Jr, Helbig TE, et al. Two- to 12-year evaluation of cementless Buechel-Pappas total hip arthroplasty. J Arthroplasty 2004;19:1017-27. [Crossref] [PubMed]
- Pappas MJ, Makris G, Buechel FF. Titanium nitride ceramic film against polyethylene: A 48 million cycle wear test. Clin Orthop Relat Res 1995;64-70. [PubMed]
- Warburton KJ, Everingham JB, Helms JL, et al. Wear testing of a canine hip resurfacing implant that uses highly cross-linked polyethylene. J Orthop Res 2018;36:1196-205. [PubMed]
- Buechel FF, Pappas MJ. Principles of Human Joint Replacement. 2nd Edition. Switzerland: Springer, 2015:184-5,223.
- Krein SW, Chao EY. Biomechanics of bipolar hip endoprostheses. J Orthop Res 1984;2:356-68. [Crossref] [PubMed]
- ASTM F561-97. Practice for Retrieval and Analysis of Implanted Medical Devices, and Associated Tissues. ASTM International, West Conshohocken, PA, 1997.
- Retrieval and analysis of surgical implant—Part 2: analysis of retrieved surgical implants. International Organization for Standardization. Geneva, Switzerland, ISO 12891, 2020.
- ASTM F2102. Standard Guide for Evaluating the Extent of Oxidation in Polyethylene Fabricated Forms Intended for Surgical Implants. ASTM International, West Conshohocken, PA, 2017.
- Hood RW, Wright TM, Burstein AH. Retrieval analysis of total knee prostheses: a method and its application to 48 total condylar prostheses. J Biomed Mater Res 1983;17:829-42. [Crossref] [PubMed]
- Ramamurti BS, Estok DM, Bragdon CR, et al. Dimensional changes in metal-backed polyethylene acetabular cups under cyclic loading. Trans ORS. J Arthroplasty 1999;24:822.
- Hollar KA, Ferguson DS, Everingham JB, et al. Quantifying wear depth in hip prostheses using 3D optical scanner. Wear 2018;394-395:195-202. [Crossref] [PubMed]
- Affatato S, Valigi MC, Logozzo S. Wear distribution of knee joint prostheses by means of 3D optical scanners. Materials 2017;10:364. [Crossref] [PubMed]
- Implants for surgery — Wear of total hip-joint prostheses — Part 1: Loading and displacement parameters for wear-testing machines and corresponding environmental conditions for test. International Organization for Standardization, Geneva, Switzerland. ISO 14242-1, 2002.
- Wear of implant materials – polymer and metal wear particles – isolation and characterization. International Organization for Standardization. Geneva, Switzerland. ISO 17853, 2010.
- Charnley J. The lubrication of animal joints in relation to surgical reconstruction by arthroplasty. Ann Rheum Dis 1960;19:10-9. [Crossref] [PubMed]
- Calton TF, Fehring TK, Griffin WL, et al. Failure of the polyethylene after bipolar hemiarthroplasty of the hip. A report of five cases. J Bone Joint Surg Am 1998;80:420-3. [Crossref] [PubMed]
- Kelly NH, Rajadhyaksha AD, Wright TM, et al. High stress conditions do not increase wear of thin highly crosslinked UHMWPE. Clin Orthop Relat Res 2010;468:418-23. [Crossref] [PubMed]
- Shen FW, Lu Z, McKellop HA. Wear versus thickness and other features of 5-Mrad crosslinked UHMWPE acetabular liners. Clin Orthop Relat Res 2011;469:395-404. [Crossref] [PubMed]
- Johnson AJ, Loving L, Herrera L, et al. Short-term wear evaluation of thin acetabular liners on 36-mm heads. Clin Orthop Relat Res 2014;472:624-9. [Crossref] [PubMed]
- Matsen Ko LJ, Pollag KE, Yoo JY, et al. Serum Metal Ion Levels Following Total Hip Arthroplasty With Modular Dual Mobility Components. J Arthroplasty 2016;31:186-9. [Crossref] [PubMed]
- Pritchett JW. Hip Replacement or Hip Resurfacing with a Highly Cross-Linked Polyethylene Acetabular Bearing: A Qualitative and Quantitative Preference Study. JB JS Open Access 2020;5:e0004.
- D’Apuzzo MR, Koch CN, Esposito CI, et al. Assessment of Damage on a Dual Mobility Acetabular System. J Arthroplasty 2016;31:1828-35. [Crossref] [PubMed]
- Loving L, Lee RK, Herrera L, et al. Wear performance evaluation of a contemporary dual mobility hip bearing using multiple hip stimulator attesting conditions. J Arthroplasty 2013;28:1041-6. [Crossref] [PubMed]
- Jaeger S, Uhler M, Schroeder S, et al. Comparison of Different Locking Mechanisms in Total Hip Arthroplasty: Relative Motion between Cup and Inlay. Materials (Basel) 2020;13:1392. [Crossref] [PubMed]
- Pritchett J. Deformation and Recovery of Thin Metal-Backed, Two-Piece Polyethylene Acetabular Resurfacing Components. J Long Term Eff Med Implants 2019;29:59-69. [Crossref] [PubMed]
- Yahia CA, Diwanji S, Ganapati M, et al. Supra acetabular bone density measurements after hip resurfacing arthroplasty at short-term followup. Hip Int 2011;21:468-74. [Crossref] [PubMed]
Cite this article as: Pritchett JW. Wear and performance of a tripolar total hip replacement. Ann Joint 2022;7:23.