Prophylactic surgical treatment using CT-based rigidity analysis vs. after the fact fracture treatment of pathologic femoral lesions
Introduction
Metastatic bone disease (MBD) is a substantial burden to patients and the healthcare system. In the United States, more than 250,000 patients have MBD with an estimated annual cost of twelve billion dollars (1). New and aggressive treatments have increased the longevity of patients with cancer, including those with a greater chance of metastasizing to bone. Consequently, the costs of orthopedic care for patients with MBD continues to rise. Further, the increased longevity of patients with MBD potentially increases their disease burden. As physicians, we have the opportunity to alleviate disease and symptomatic burden on these patients but are increasingly asked to do so while simultaneously alleviating the burden on our health care system.
Orthopedic care of MBD patients usually includes either operative treatment of pathologic fractures or impending fractures. Prophylactic surgical treatment (PST) is meant to prevent fracture, limit disability, and shorten hospitalization compared to internal fixation after fracture (AF). Patients who are treated prophylactically may be better mentally prepared for surgery and the recovery process and spared the trauma of the fracture event. Prophylactic surgery is often simpler and theoretically is associated with fewer complications and better outcomes. To some extent, published literature bears this out, with the possible exception of venous thromboembolic disease (1). Advantages attributed to prophylactic stabilization compared to treatment after pathologic fractures include lower blood loss, less transfusions, lower rates of urinary tract infections, lower rates of discharge to rehabilitation facility compared to home, decreased direct and total costs, shortened hospital stay, increased incidence of independent ambulation, and decreased post-operative morbidity (1-5).
However, the purported benefits of PST have been based upon poor or absent definitions of impending pathologic fractures, including Mirels scoring. Mirels scoring has been shown to have low specificity for predicting impending fracture, so studying groups of patients defined by Mirels to have impending pathologic fractures is inherently biased to include a relatively high percentage of patients who do not truly have impending fractures as defined by more accurate techniques such as CT-based structural rigidity analysis (CTRA) (6-8). CT-based structural rigidity analysis has been shown to be a better predictor of impending pathological fractures than the Mirels score in long bone lesions affected by disseminated malignancy (6-8). The current study uses CTRA to more precisely define a group of patients with impending fractures due to disseminated malignancies affecting the femur and then to compare cost and clinical benefits of this more restrictive group of impending pathologic fractures treated with PST compared to patients with pathologic fractures treated AF. Three major questions are evaluated in this more restrictive PST group compared to AF. (I) Is there an advantage of PST in clinical endpoints of estimated blood loss, number of blood transfusions, length of hospital stay, or survival? (II) Is there an advantage of PST in discharge disposition (home versus rehab)? (III) Are costs lower with PST? The hypotheses are that there are clinical, discharge, and cost benefits to PST (for CTRA defined impending fractures) when compared to AF surgery.
We present the following article in accordance with the STROBE reporting checklist (available at https://aoj.amegroups.com/article/view/10.21037/aoj-20-92/rc).
Methods
We performed an institutional review board-approved study of patients treated by the orthopedic oncology division at SUNY Upstate Medical University Department of Orthopedic Surgery. Two cohorts were compared. The PST cohort had been enrolled and treated by the senior author between February 2009 and December 2016 in a longitudinal study of impending pathologic fractures evaluated for a multicenter study and were treated during that same time period (Figure 1). The study sponsored by the Musculoskeletal Tumor Society evaluated the diagnostic accuracy of CT-based structural rigidity analysis (CTRA) (6-8). The AF cohort was identified retrospectively by searching our office database for ICD codes related to metastatic carcinoma, myeloma, or lymphoma (Addendum A) and combined with CPT codes (Addendum B) for internal fixation and or endoprosthetic treatment of those patient diagnoses from December 2011 until February 2016 (Figure 2). The collection of data prior to 2011 was complicated by the unavailability of electronic medical records prior to that date. Chart review was done to confirm the ICD diagnosis and CPT code, and only those patients confirmed to have metastatic carcinoma, myeloma, or lymphoma involving either femur for which they underwent either open reduction with internal fixation (ORIF) or resection and endoprosthetic arthroplasty reconstruction (RR) were included. Only those patients treated by the PI were included. All patients identified in the retrospective search after exclusions were included in the study over the period designated. The study was conducted in accordance with the Declaration of Helsinki (as revised in 2013). The study was approved by the Institutional Review Board at SUNY Upstate Medical University (IRB #00000391). Informed consent was taken from all individual participants in the prospectively enrolled cohort and individual consent for the retrospective cohort was waived.
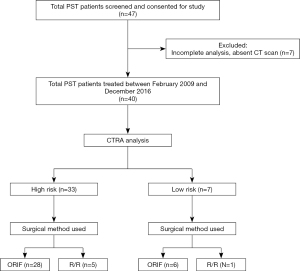
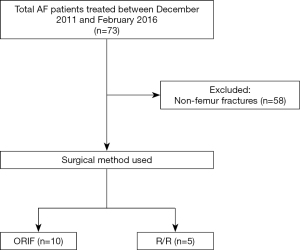
A total of 55 patients after exclusions were identified and treated surgically for metastatic femoral lesions and were divided into three groups for the purpose of this study. The AF cohort, designated as group I, included 15 (27% of 55) patients identified by retrospective chart review who presented with pathologic fracture and were treated surgically after the pathologic fracture occurred. The PST cohort consisted of 40 total patients and included groups II and III. Group II (PST-high) consisted of 33 (60% of 55) patients identified as high risk for impending pathologic femur fracture by CTRA who then underwent PST. Group III (PST-low) included 7 (13% of 55) patients who underwent PST despite low impending pathologic fracture risk by CTRA definition, since surgeon discretion in treatment choice was allowed under the protocol for the longitudinal MSTS study of CTRA (6-8). Surgical treatment in all groups included either open reduction and internal fixation (ORIF) or resection/reconstruction with an endoprosthesis (RR). Demographic information, comorbidities, and clinical variables of interest were collected by retrospective chart review; cost data was collected by collaboration with our hospital financial personnel (office of the Chief Financial Officer).
With respect to co-morbidities, survival probability and disease extent, the scoring system by Janssen et al. was used (9) (Table 1). Additional comorbidities were defined using a modified Charlson Comorbidity Index including conditions such as diabetes mellitus, liver disease, acquired immune deficiency syndrome (AIDS), chronic kidney disease (CKD) (moderate-severe), congestive heart failure (CHF), myocardial infarction (MI), chronic obstructive pulmonary disease (COPD), peripheral vascular disease (PVD), cerebrovascular accident (CVA) or transient ischemic attack (TIA), dementia, hemiplegia, connective tissue disease, and peptic ulcer disease. Primary tumors with poor prognosis included lung and others, but excluded breast, kidney, prostate, thyroid, myeloma, and lymphoma (10-12). Visceral metastases included sites of lung, brain, or liver (10). Preoperative hemoglobin levels were accepted when they were recorded within 7 days preop. According to this assessment system, only those variables shown to have been statistically significantly associated with decreased likelihood of survival after surgical treatment were used (10). A poor prognosis was defined as a total score of 6–10, an intermediate prognosis as 3–5, and a good prognosis as 0–2.
Table 1
Variable | Points |
---|---|
Age, years | |
≥65 | 1 |
<65 | 0 |
Additional comorbidities* | 1 |
BMI, kg/m2 | |
<18.5 | 2 |
18.5–30 | 0 |
>30 | 1 |
Primary tumor with poor prognosis* | 2 |
Multiple bone metastases | 1 |
Visceral metastases* | 2 |
Preoperative HGB, g/dL | |
>10 | 0 |
≤10 | 1 |
Weighted score for non-continuous factors assigned by rounding its hazard ratio to the nearest integer. Continuous variables (age, preoperative hemoglobin levels) were dichotomized. *, see text for detailed methods. A poor prognosis was defined as a total score of 6–10, an intermediate prognosis as 3–5, and a good prognosis as 0–2. Table is adapted from one published by Janssen et al. and permission for using the above table was granted by Wolters Kluwer Health, Inc. (9). BMI, body mass index; HGB, hemoglobin.
Clinical outcome variables included estimated blood loss (EBL), transfusions given (intra-operatively and post-operatively), length of hospital stay (LOS), discharge disposition (home, rehabilitation center, hospice, or morgue), and survival duration. Patients who expired post-operatively in the hospital were excluded from LOS analysis to avoid unfairly biasing the LOS to lower values for those groups including those patients. Cost data included direct and total costs. Direct costs included costs of labor, medical supplies, and any surgical implants. Indirect cost makes up the difference between direct and total costs and includes secondary but necessary costs, such as administrative services and utility costs. No out of hospital costs were collected, so costs of rehabilitation, skilled nursing facilities, hospice, home care, and outpatient physical therapy were not reflected in this data.
Statistical analysis
Statistical significance was assessed by single-factor ANOVA for comparison of each of the quantitative endpoints between the patient groups. Significance in variation of co-morbidity and discharge disposition were assessed using a chi-square test. Results are expressed as mean values. For each, statistical significance was accepted at P≤0.05.
Results
Clinical characteristics of the three different groups are shown in Table 2. With respect to demographics and clinical duration follow-up, no significant differences were found between treatment groups (Table 2). The distribution of underlying primary cancers was also similar between groups (Figure 3). No significant difference was found in co-morbidity between groups (Table 2, Figure 4).
Table 2
Variable | Group I | Group II | Group III | P value |
---|---|---|---|---|
Age (years) | 62.5 [6–87] | 64.5 [45–82] | 69.1 [51–88] | 0.534 |
Sex | 0.772 | |||
Male | 8 (53%) | 14 (42%) | 3 (43%) | |
Female | 7 (47%) | 19 (58%) | 4 (57%) | |
Anatomic site* | 0.298 | |||
Epiphysis | 1 (7%) | 7 (21%) | 1 (14%) | |
Metaphysis | 11 (73%) | 20 (61%) | 6 (86%) | |
Diaphysis | 6 (40%) | 14 (42%) | 2 (29%) | |
Follow up duration (weeks)# | 35.7 | 49.3 | 10.7 | 0.232 |
Type of procedure | 0.318 | |||
ORIF | 10 (67%) | 28 (85%) | 6 (86%) | |
R/R | 5 (33%) | 5 (15%) | 1 (14%) | |
Prognosis based on Jansen Co-Morbidity Index Score§ | – | |||
Poor (6–10 points) | 4 | 3 | 0 | |
Intermediate (3–5 points) | 6 | 13 | 6 | |
Good (0–2 points) | 4 | 13 | 0 | |
Average score | 4.21 | 3.28 | 4 | 0.192 |
*, Group I: AF surgical treatment; Group II: PST-High surgical treatment (prophylactic surgical treatment with high risk estimated by CTRA); Group III: PST-Low (PST with low risk estimated by CTRA). Anatomic site: 3 (30%) of Group I, 6 (18%) of Group II, and (29%) of Group III had lesions through 2 anatomic sites; 1 (3%) of Group II had lesions extending through 3 sites. #, follow-up duration: 3 patients in Group II and 1 patient in Group III had inadequate records to evaluate. §, prognosis based on Jansen Co-Morbidity Index Scores: 1 patient in Group 1, 4 patients in Group II, and 1 patient in Group III had inadequate records to evaluate. ORIF, open reduction and internal fixation; R/R, resection and reconstruction.
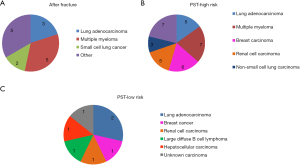
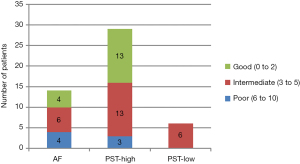
Is there an advantage of PST compared to AF in terms of clinical endpoints such as estimated blood loss, number of blood transfusions, length of hospital stay, and survival?
The number of transfusions given was lowest and nearly equal in groups II and III, but there was no statistically significant difference with group I (despite being nearly twice that of group II on average) (Table 3). Estimated blood loss was generally low, resulting in no statistically significant difference. Similarly, there were no statistically significant differences in length of stay between the groups. However, survival showed statistically significant differences favoring group II (PST-High) (Table 3).
Table 3
Variable | Group I | Group II | Group III | P value |
---|---|---|---|---|
LOS (days) | 9.5 | 9.5 | 8.3 | 0.966 |
EBL (mL) | 215 | 221 | 250 | 0.845 |
Blood transfusion (units PRBC) | 1.93 | 0.94 | 1 | 0.398 |
Disease status at follow-up | <0.001 | |||
Alive with disease | 3 (20%) | 25 (83%) | 3 (50%) | |
Dead of disease | 11 (73%) | 5 (17%) | 2 (33%) | |
Dead of complications | 1 (7%) | 0 (0%) | 1 (17%) | |
Direct cost (USD) | 18,836.53 | 16,093.57 | 17,171.93 | 0.758 |
Total cost (USD) | 31,997.24 | 27,357.16 | 29,668.59 | 0.778 |
Disease status at follow-up: 3 patients in Group II and 1 patient in Group III had inadequate records to evaluate. LOS, length of stay; EBL, estimated blood loss; PRBC, packed red blood cells; USD, United States dollars.
Is there an advantage of PST compared to AF in discharge disposition (home versus rehab)?
Discharge disposition showed statistically significant differences between groups (P=0.012, global) (Figure 5). Discharge to home was highest in group II (PST-High) (76%) and lowest in group I (AF) (27%). Discharge to rehab was lowest in group II (PST-High) (24%) and highest in group I (AF) (47%). There were no discharges to hospice or morgue in group II (PST-High), while both occurred in group I (AF). Statistically, discharge disposition for group I was significantly different from group II (P=0.002) as well as groups II and III combined (P=0.006) but not different from group III alone (P=0.511). Group II discharge disposition was statistically significantly different from group I (P=0.002), group III (P=0.042), and groups I and III combined (0.003).
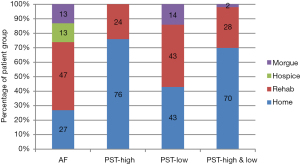
Are costs lower with PST compared to surgery AF?
Mean direct and total costs were highest in Group I (AF) ($18,837 and $31,997, respectively) and lowest in Group II (PST-High) ($16,094 and $27,357) (Table 1). However, these differences between the groups did not reach statistical significance for either direct or total costs.
Discussion
Prior studies showing benefits to PST over those treated AF may be criticized for including patients in the PST groups that were not truly at risk for pathologic fracture and therefore not actually indicated for surgical intervention. These conditions may have artificially exaggerated advantages of PST over AF treatment because PST groups in the past likely included patients for which surgery was not actually indicated from a purely biomechanical basis. The lesions in those patients may have been less compromised than those lesions that are truly at risk for fracture by more strict criteria. This study is unique in more precisely defining the group of PST patients by using CTRA, making it more likely that the included patients truly had impending pathologic fractures and needed prophylactic surgery. Therefore, narrowing the PST group in this way allows a more relevant comparison with the AF group. Hence, it is expected that if this more restricted group of patients defined to have “impending pathologic fractures” does show a benefit, then those demonstrable beneficial results can be fairly used to support the findings of other studies that have shown beneficial results of PST. The results of this study succeed in doing that for some variables (discharge disposition and overall survival) while falling short with others (transfusions, EBL, LOS) from a statistical perspective.
The definition of impending pathologic fractures continues to evolve over time. In the past, Harrington and others offered level IV and V evidence supporting numerous single variables purported to signify increased risk of fracture. These definitions have largely been shown to be unreliable. More recently, criteria such as those of Mirels and Carnesale, as well the “L-cort” definition have been used widely (13-15). Evaluation of these criteria have drawn two major conclusions. First, in terms of accuracy, comparison between these techniques shows that the L-cort definition of impending pathologic fracture (largest axial cortical involvement >30 mm) has greater accuracy than the Carnesale definition (>50% cortical involvement or >3 cm size), and both are more accurate than Mirels (a 12 point scale based on four criteria—site, size, type of bone involvement, and degree of pain—with 9 points being the suggested definition of impending fracture (16,17). Second, and more importantly, while all three of these modern criteria are highly reliable and reproducible with high sensitivity and high negative predictive values, they are low in specificity, positive predictive values, and hence overall accuracy (18-20).
The low accuracy of these simple techniques continues to be the impetus behind the development of new techniques for prediction of impending pathologic fractures. Hence, the CT based techniques of CTRA and Fine Element Method (FEM) have evolved and been applied to this setting of long bone lesions due to metastatic disease, myeloma, and lymphoma (6-8,21). Direct comparison with Mirels criteria shows superior specificity, positive predictive value, and accuracy while maintaining very high sensitivity and negative predictive value (6-8,21). Other new techniques, including SPECT-CT, FDG-PET, and machine learning are developing on the horizon (22-24).
Prior articles evaluating the benefits of prophylactic stabilization compared to fixation after pathologic fractures have suggested benefits to prophylactic internal fixation over fixation of pathologic fractures (1-5). Ward et al., in describing the senior author’s 12-year experience with 97 prophylactically fixed lesions compared to 85 pathologic fractures, showed statistically significant benefits to prophylactic fixation in terms of estimated average blood loss, length of hospital stay, discharge to home vs. rehabilitation, resumption of support free ambulation, and ability to utilize intramedullary fixation rather than more expensive endoprostheses (5). Behnke et al. demonstrated the devastating effects of pathologic fractures on same admission inpatient morbidity and mortality by querying the 371,163 entries for pathologic fractures in the Nationwide Inpatient Sample between 2002 and 2013 (2). In their series, they demonstrated statistically significantly lower blood transfusion, hemorrhage/hematoma, acute post-operative anemia, acute renal failure, acute myocardial infarction, and mortality in patients treated with impending fractures. No difference was demonstrated in either length of stay or cost (2). In a similar query of the Nationwide Inpatient Sample for the 10-year period from 2002 through 2011 with a focus on venous thromboembolic disease, Aneja et al. showed that while there was a lower incidence of transfusion, lower risk of urinary tract infection and higher likelihood of discharge home, patients undergoing prophylactic fixation were in fact at a higher risk of both deep venous thrombosis and pulmonary embolism (1). Blank et al. compared 21 patients with impending fractures to 19 patients treated after pathologic fractures with an emphasis on the potential economic advantages of treating prior to fracture (3). They showed mean lower total and direct costs with prophylactic fixation as well as shorter length of stay. The estimated mean reduction in total cost was USD 21,000 and direct cost reduction of USD 12,000 with prophylactic stabilization (3). These figures compare to reductions of approximately USD 4,000 and USD 3,000, respectively, in the current study of CTRA defined PST treatment. With the decreased magnitude of differences in the current study, statistical significance was not able to be proven. Utilizing the National Surgical Quality Improvement Program (NSQIP) database, McLynn and colleagues also showed the advantage of decreased need for transfusion in the prophylactic patient population compared to after fracture treatment even after correction for patient characteristics (4).
The major flaw in each of these five papers, however, is that they failed to provide consistent definitions of the included impending pathologic fractures (1-5). Hence, while they appear at face value to show at least some advantages to prophylactic fixation, there is little evidence to support the contention that the patients in the “impending fracture” groups in each of these papers were indeed impending. Basically, the reader of these studies is asked to accept at face value the suggestion that because a procedure was coded as being a prophylactic procedure that the bone operated on was in fact at significantly increased risk of fracture. Given the now well-established inability of clinicians to accurately predict fracture risk, even with tools such as Mirels, this suggestion has to be considered contentious. The current study is the first to attempt to address this major flaw.
The two positive findings supporting advantages to prophylactic fixation in this paper are the improvement in discharge home over discharge to rehab facilities and the improvement in survival. The most impactful finding was the significant difference in discharge disposition observed between groups favoring PST over AF. This corresponds to the findings of others that a higher percentage of patients with prophylactic fixation were able to be discharged home (1,5). Not all studies that examined this endpoint were able to demonstrate any statistical advantage in discharge, making the current findings all the more important (2,3). We believe this paper provides the best objective evidence supporting the advantage of PST in achieving discharge home due to the aforementioned unique use of CTRA to define impending fractures more precisely. Further, this finding may have a bearing on cost as well. This paper reports only on in-hospital costs. However, since overall cost, when it includes the much discussed 90-day period post-operatively, is heavily affected by discharge and negatively impacted by discharge to rehabilitation/skilled nursing facilities, the overall combined inpatient and outpatient cost is likely dramatically higher, on average, for AF subjects when compared to PST subjects. Without more detailed analysis of those out of hospital costs, we are unable to prove the cost-effectiveness of PST over AF.
One unexpected finding was the statistically significant difference in duration of survival between groups even at this relatively short duration follow-up. This occurred in the absence of statistically significant differences in co-morbidities between groups which was determined using the scoring system by Janssen et al., as discussed previously (9). However, it agrees with the findings of the Nationwide Inpatient Study evaluation (2). This finding in our paper may represent a similar early but more dramatic difference with an accelerated decline in these patients similar to that observed by Behnke et al. (2). Conversely, whether the improved survival with PST is life-prolonging or simply reflects unproven lesser co-morbidities in the PST group II subjects cannot be known for sure. In the NSQIP based study reported by McLynn, after correction for patient features, the advantages in terms of survival for the prophylactic group were non-existent (4). The fact that survival in Group III was intermediate between groups AF and Group II despite no increase in baseline co-morbidities likely stemmed from the disease progression seen in two and the death due to a complication in another subject in that small group of seven subjects. It is pertinent to note that all patients in Group III were intermediate in co-morbidity, but with the very small number of patients in this group, it is difficult to draw definitive conclusions.
Trends for cost (direct and total) and transfusion favored PST over AF, as they have in earlier reports, but in this study, they lacked significance. Similarly, we showed NS differences in LOS or EBL. We included patients who underwent either ORIF or R/R both for AF and PST. Arthroplasty reconstruction following resection is a higher cost procedure than ORIF in general, so the inclusion of arthroplasty may have increased in-hospital direct and total costs for Group I in this study, as arthroplasty was used in 33% of Group I subjects compared to 15% of Group II subjects.
Limitations of this study include the small number of patients, which limits subgroup analysis, and the inherent weaknesses of a retrospective cohort study (AF, group I) even though the other groups consisted of longitudinally collected data. Group III (PST-low) was especially small (n=7), further exacerbating difficulties in showing statistical differences with that group even when they appeared to possibly be present (such as the possibility of higher co-morbidity in Group III compared to Group II (PST-high). It is important to again note that all patients included in this study were treated by the same surgeon. This choice was intentional, as it limits intraoperative variability in order to better compare study groups. It allows statistically significant differences in outcome measures to be more strongly attributed to differences in the treatment options themselves, rather than variability of surgical choices or preferences made by different surgeons. That being said, choosing to include patients all treated by the same surgeon can limit the generalizability of the study results. The inclusion of R/R within the PST groups may have contributed to increased relative costs, blood loss and length of stay in Groups II and III, but R/R treatment was also included in Group I (AF). The relatively small number of subjects in the AF group may have been contributed to by numerous factors, including referral patterns within our community between private and academic centers, patient care and referral patterns by radiation oncologists, and enrollment in the prophylactic screening MSTS study. The latter factor may have culled some of the subjects that would have otherwise fractured. Finally, with respect to costs, our data was focused only on in-hospital direct and total costs. Later costs of rehabilitation facilities, skilled nursing facilities, hospice, home care, and physical therapy during the 90-day post-discharge period, were not captured. Hence, we were unable to demonstrate the likely overall cost savings that benefits PST over AF given that the latter is associated with a statistically significantly increased percentage of discharge to rehab vs. home. Differences in costs for total joint patients depending upon discharge disposition are well established, and they no doubt apply to this patient population as well, but they were not collected for individuals in this study (25).
Conclusions
These findings show the potential for improved discharge disposition and survival benefit of prophylactic surgery for metastatic lesions of the femur when compared to waiting for fractures to occur first before treating surgically afterwards in a patient population that has been specifically restricted to an accurate and clinically verified CT-based definition of impending pathologic fracture. Further prospective studies evaluating these patients should strive to utilize tools that accurately define impending pathologic fractures.
Acknowledgments
The authors wish to acknowledge the Chief Financial Officer at our institution, Stuart Wright, along with his office staff, who were responsible for providing the financial data included in this paper. We acknowledge the contribution of Sri Pinnamaneni, MD, in contributing coding information from a related study of pathologic fracture patients utilized in the screening for current patients from the after-fracture cohort in this study. Furthermore, we acknowledge the developers of the CT-based rigidity analysis technique with whom we have frequently collaborated, particularly Brian Snyder, MD and Ara Nazarian, PhD. It is from their laboratory at the Beth Israel Deaconess Hospital Biomechanics Laboratory at Harvard University that we have obtained the CTRA analyses for this study. Their continued work in sponsoring the ongoing larger multi-institutional study is acknowledged. Finally, we graciously acknowledge the members of the Musculoskeletal Tumor Society who, by way of funding from the Orthopaedic Research Society and through their own contributions of donations, patients (albeit not included in this study), and time, have made this work possible.
Funding: Tina Craig reports grants from Orthopedic Research and Education Foundation and from Carol Baldwin Breast Cancer Research Foundation during the conduct of the study. Dr. TAD reports grants from Musculoskeletal Tumor Society and Orthopedic Research and Education Foundation, grants from Carol Baldwin Breast Cancer Research Foundation, during the conduct of the study; grants from National Institutes Of Health (NIH), grants from Stryker, Inc, grants from Wright Medical, Inc, support from William Smythe Cancer Fund, personal fees from Bone Support Orthopedic Physician Advisory Board, grants from Jim and Juli Boeheim Pediatric Cancer Graft Support, other from David G. Murray Endowed Professorship, outside the submitted work.
Footnote
Provenance and Peer Review: This article was commissioned by the Guest Editor (Rui Yang) for the series “Bone Metastasis” published in Annals of Joint. The article has undergone external peer review.
Reporting Checklist: The authors have completed the STROBE reporting checklist. Available at https://aoj.amegroups.com/article/view/10.21037/aoj-20-92/rc
Data Sharing Statement: Available at https://aoj.amegroups.com/article/view/10.21037/aoj-20-92/dss
Peer Review File: Available at https://aoj.amegroups.com/article/view/10.21037/aoj-20-92/prf
Conflicts of Interest: All authors have completed the ICMJE uniform disclosure form (available at https://aoj.amegroups.com/article/view/10.21037/aoj-20-92/coif). The series “Bone Metastasis” was commissioned by the editorial office without any funding or sponsorship. TAD declares that other than the musculoskeletal tumor society funding, which has long since been completed, and ongoing related work examining other aspects of fracture risk prediction, none of the sources of funding represent related sources or conflicts. The authors have no other conflicts of interest to declare.
Ethical Statement:
Open Access Statement: This is an Open Access article distributed in accordance with the Creative Commons Attribution-NonCommercial-NoDerivs 4.0 International License (CC BY-NC-ND 4.0), which permits the non-commercial replication and distribution of the article with the strict proviso that no changes or edits are made and the original work is properly cited (including links to both the formal publication through the relevant DOI and the license). See: https://creativecommons.org/licenses/by-nc-nd/4.0/.
References
- Aneja A, Jiang JJ, Cohen-Rosenblum A, et al. Thromboembolic Disease in Patients with Metastatic Femoral Lesions: A Comparison Between Prophylactic Fixation and Fracture Fixation. J Bone Joint Surg Am 2017;99:315-23. [Crossref] [PubMed]
- Behnke NK, Baker DK, Xu S, et al. Risk factors for same-admission mortality after pathologic fracture secondary to metastatic cancer. Support Care Cancer 2017;25:513-21. [Crossref] [PubMed]
- Blank AT, Lerman DM, Patel NM, et al. Is Prophylactic Intervention More Cost-effective Than the Treatment of Pathologic Fractures in Metastatic Bone Disease? Clin Orthop 2016;474:1563-70. [Crossref] [PubMed]
- McLynn RP, Ondeck NT, Grauer JN, et al. What Is the Adverse Event Profile After Prophylactic Treatment of Femoral Shaft or Distal Femur Metastases? Clin Orthop 2018;476:2381-8. [Crossref] [PubMed]
- Ward WG, Holsenbeck S, Dorey FJ, et al. Metastatic disease of the femur: surgical treatment. Clin Orthop 2003;S230-44. [Crossref] [PubMed]
- Damron TA, Nazarian A, Entezari V, et al. CT-based Structural Rigidity Analysis Is More Accurate Than Mirels Scoring for Fracture Prediction in Metastatic Femoral Lesions. Clin Orthop 2016;474:643-51. [Crossref] [PubMed]
- Nazarian A, Entezari V, Villa-Camacho JC, et al. Does CT-based Rigidity Analysis Influence Clinical Decision-making in Simulations of Metastatic Bone Disease? Clin Orthop 2016;474:652-9. [Crossref] [PubMed]
- Nazarian A, Entezari V, Zurakowski D, et al. Treatment Planning and Fracture Prediction in Patients with Skeletal Metastasis with CT-Based Rigidity Analysis. Clin Cancer Res 2015;21:2514-9. [Crossref] [PubMed]
- Janssen SJ, van der Heijden AS, van Dijke M, et al. 2015 Marshall Urist Young Investigator Award: Prognostication in Patients With Long Bone Metastases: Does a Boosting Algorithm Improve Survival Estimates? Clin Orthop 2015;473:3112-21. [Crossref] [PubMed]
- Deyo RA, Cherkin DC, Ciol MA. Adapting a clinical comorbidity index for use with ICD-9-CM administrative databases. J Clin Epidemiol 1992;45:613-9. [Crossref] [PubMed]
- Quan H, Parsons GA, Ghali WA. Validity of information on comorbidity derived from ICD-9-CCM administrative data. Med Care 2002;40:675-85. [Crossref] [PubMed]
- Voskuijl T, Hageman M, Ring D. Higher Charlson Comorbidity Index Scores are associated with readmission after orthopaedic surgery. Clin Orthop 2014;472:1638-44. [Crossref] [PubMed]
- Carnesale P. Malignant tumours of bone. In: St Canale JB, editor. Campbell’s Operative Orthopaedics. 10th ed. Amsterdam: Elsevier, 200:551.
- Mirels H. Metastatic disease in long bones: a proposed scoring system for diagnosing impending pathologic fractures. Clin Orthop 1989;256-64. [Crossref] [PubMed]
- van der Linden YM, Kroon HM, Dijkstra SP, et al. Simple radiographic parameter predicts fracturing in metastatic femoral bone lesions: results from a randomised trial. Radiother Oncol 2003;69:21-31. [Crossref] [PubMed]
- El-Husseiny M, Coleman N. Inter- and intra-observer variation in classification systems for impending fractures of bone metastases. Skeletal Radiol 2010;39:155-60. [Crossref] [PubMed]
- Van der Linden YM, Dijkstra PD, Kroon HM, et al. Comparative analysis of risk factors for pathological fracture with femoral metastases. J Bone Joint Surg Br 2004;86:566-73. [Crossref] [PubMed]
- Damron TA, Morgan H, Prakash D, et al. Critical evaluation of Mirels' rating system for impending pathologic fractures. Clin Orthop 2003;S201-7. [Crossref] [PubMed]
- Evans AR, Bottros J, Grant W, et al. Mirels' rating for humerus lesions is both reproducible and valid. Clin Orthop 2008;466:1279-84. [Crossref] [PubMed]
- Mac Niocaill RF, Quinlan JF, Stapleton RD, et al. Inter- and intra-observer variability associated with the use of the Mirels' scoring system for metastatic bone lesions. Int Orthop 2011;35:83-6. [Crossref] [PubMed]
- Goodheart JR, Cleary RJ, Damron TA, et al. Simulating activities of daily living with finite element analysis improves fracture prediction for patients with metastatic femoral lesions. J Orthop Res 2015;33:1226-34. [Crossref] [PubMed]
- Riaz S, Bashir H, Niazi IK, et al. 99mTc MDP SPECT-CT-Based Modified Mirels Classification for Evaluation of Risk of Fracture in Skeletal Metastasis: A Pilot Study. Clin Nucl Med 2018;43:e180-3. [Crossref] [PubMed]
- Ulaner GA, Zindman AM, Zheng J, et al. FDG PET/CT Assesses the Risk of Femoral Pathological Fractures in Patients With Metastatic Breast Cancer. Clin Nucl Med 2017;42:264-70. [Crossref] [PubMed]
- Wang Z, Wen X, Lu Y, et al. Exploiting machine learning for predicting skeletal-related events in cancer patients with bone metastases. Oncotarget 2016;7:12612-22. [Crossref] [PubMed]
- Lavernia CJ, D’Apuzzo MR, Hernandez VH, et al. Postdischarge costs in arthroplasty surgery. J Arthroplasty 2006;21:144-50. [Crossref] [PubMed]
Cite this article as: DeHority K, Craig T, Damron TA. Prophylactic surgical treatment using CT-based rigidity analysis vs. after the fact fracture treatment of pathologic femoral lesions. Ann Joint 2022;7:12.