Deletion of tuberous sclerosis complex 1 (TSC1) in limb bud mesenchyme causes chondrodysplasia and severe knee joint osteoarthritis in mice
Introduction
The tumor suppressor genes, tuberous sclerosis complex 1 (TSC1) and TSC2, encode hamartin and tuberin, respectively, which are key upstream negative regulators of the mechanistic target of rapamycin complex 1 (mTORC1) (1-3). Mutations of TSC1 or TSC2 induce abnormal activation of mTORC1 that contributes to the development of the genetic disorder TSC, which is characterized by benign hamartomas in multiple organ systems (4,5). There is also frequent involvement of the skeletal system in TSC such as sclerotic bone lesions with unknown mechanisms. Patients with tuberous sclerosis due to mutation in TSC1/2 present with sclerotic and lytic bone changes (5-9).
The mTOR complex functions as a master regulator of cell proliferation, growth, metabolism and survival, in response to nutrients, growth factors, and stresses. mTOR forms two larger protein complexes, mTOR complex 1 (mTORC1) and mTOR complex 2 (mTORC2) (1-3). mTORC1 is a sensitive target of rapamycin that controls cell growth mainly by stimulating protein synthesis, achieved via phosphorylation of ribosomal S6 protein kinase (S6K) and 4E-binding protein-1 (4E-BP1), two key factors in translation initiation (1-3).
mTORC1 signaling has recently emerged as a critical regulator of skeletal growth and bone formation (10-15). Disruption of mTORC1 signaling through deletion of either mTOR or the associated protein raptor diminishes embryonic skeletal growth associated with severe delays in chondrocyte hypertrophy and bone formation (10). We have shown that hyperactivation of mTORC1 via Tsc1 gene deletion in chondrocytes (col2a1-Cre) causes uncontrolled cell proliferation, and blockage of chondrocyte terminal maturation in the growth plate, resulting in chondrodysplasia in mice (14). Moreover, we found that activation of mTORC1 in preosteoblasts (Osx-Cre) by deletion of Tsc1 produced immature woven bone in mice due to excess proliferation but impaired differentiation and maturation of the cells (15). However, the role of TSC in limb and joint development is unknown, and the effects of limb bud mesenchyme cell-specific mTORC1 activation on limb and joint development have not been reported.
In this study, to gain insights into the specific role of TSC1 in limb development, we generated mice with Tsc1 gene deletions in limb bud mesenchyme by crossing Tsc1flox/flox mice with Prx1-cre mice. We found that deletion of TSC1 and activation of mTORC1 in limb bud mesenchyme causes chondrodysplasia and severe knee joint osteoarthritis (OA) in mice. It is suggested that mesenchymal TSC1 is essential for normal limb and joint development and function.
We present the following article in accordance with the ARRIVE reporting checklist (available at http://dx.doi.org/10.21037/aoj-17-21).
Methods
Mice
Tsc1 floxed mice (Jax #005680) and Prx1-Cre mice (Jax #005584) were purchased from the Jackson Laboratories (Bar Harbor, ME, USA). To generate mice with a limb bud mesenchyme-specific Tsc1 deletion, mice of a Tsc1 floxed line were crossed with Prx1-Cre mice. Mice at different ages were used regardless of gender. We performed genotyping using genomic DNA isolated from tail biopsies, and the primers used were: Prx1-Cre primers (forward 5'-TCCAATTTACTGACCGTACACCAA-3'; reverse 5'-CCTGATCCTGGCAATTCGGCTA-3') and TSC1-loxp primers (forward 5'-GTCACGACCGTAGGAGAAGC-3'; reverse 5'-GAATCAACCCCACAGAGCAT-3') (6). The specificity of recombination was examined by PCR using regions flanking the floxed allele. All experiments were performed in accordance and approved by the Southern Medical University Experimental Animal Welfare and Ethics Committee.
Preparation of decalcified sections, histochemistry and immunohistochemistry (IHC)
Bone tissues dissected from the mice were fixed using 4% paraformaldehyde in phosphate-buffered saline (PBS) at 4 °C for 24 hours and then decalcified in 15% ethylenediaminetetraacetic acid (EDTA) (pH 7.4) at 4 °C for 14 days. The tissues were embedded in paraffin or optimal cutting temperature (OCT) compound (Sakura Finetec USA, Torrance, CA, USA) and 2–5 µm sagittal-oriented sections were prepared for histologic analyses using hematoxylin and eosin (H&E) and safranin O/fast green staining. For IHC, we incubated sections with primary antibodies which recognized mouse phosphor-S6 ribosomal protein (Ser235/236) (1:1,000 for WB; 1:100 for IHC, CST, #2211) at 4 °C overnight. All sections were observed and photographed on an Olympus BX51 microscope (Tokyo, Japan).
Western blotting
Tissues were lysed in 2% sodium dodecyl sulfate (SDS) with 2 M urea, 10% glycerol, 10 mM Tris-HCl (pH 6.8), and 10 mM phenylmethylsulfonyl fluoride. The lysates were centrifuged and the supernatants were removed, separated by SDS polyacrylamide gel electrophoresis (SDS-PAGE) and blotted onto a nitrocellulose (NC) membrane (Bio-Rad Laboratories, Hercules, CA, USA). The membrane was then analyzed using specific antibodies and visualized by enhanced chemiluminescence (ECL kit, Amersham Biosciences, GE Healthcare, Little Chalfont, UK).
Micro-computed tomography (micro-CT) analysis
Quantitative analysis was performed in mice femora at 20 µm resolution on a micro-CT Scanner (Scanco Medical, Bassersdorf, Switzerland). Briefly, scanning was performed from the lower growth plate of each femur and extended proximally for 300 slices. We began morphometric analysis with the first slice in which the femoral condyles were fully merged and extended for 100 slices proximally. The three-dimensional (3D) structure and morphometry were constructed and analyzed for bone volume/total volume (BV/TV), bone mineral density (BMD) (mg HA/mm3), trabecular number (Tb.N) (mm−1), trabecular thickness (Tb.Th) (mm) and trabecular spacing (Tb.Sp) (mm).
Statistical analysis
All results are expressed as mean ± SEM. Student’s t-tests were used to calculate P values Statistical significance was defined as P<0.05.
Results
Generation of mice with TSC1 deletion in limb bud mesenchyme
We first generated conditional knockout mice with TSC1 deletion limited to the limb bud mesenchymal cells by crossing floxed Tsc1 mice with Prx1-Cre mice. We selected Prx1-creTG/+;Tsc1flox/flox (hereafter referred to as TSC1KO) mice and Prx1-CreTG/+;TSC1+/+ (hereafter referred to as CONTROL) for detailed analysis. The deleted allele was amplified by PCR from tissue samples of TSC1KO and CONTROL mice (Figure 1A). Immunohistochemical staining of distal femur sections showed a dramatic increase in P-S6 (S235/236) in 8-week-old TSC1KO mice. Specific enhancement of P-S6 (S235/236) in chondrocytes from the proliferation and hypertrophic zones (PZ and HZ) was observed in the growth plate of TSC1KO mice (Figure 1B). To examine the recombination in osteoblasts, osteoblasts were obtained from newborn TSC1KO mice and their littermate controls. Western blotting analysis demonstrated the efficient deletion of TSC1 in osteoblasts with activating P-S6 (S235/236) (Figure 1C). These results indicated that the Tsc1 gene had been specifically deleted in mesenchymal cells, chondrocytes and osteoblasts in the limbs of TSC1KO mice.
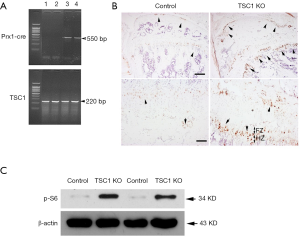
Deletion of TSC1 in limb mesenchyme results in dwarfism in mice
Although TSC1KO mice were born at normal Mendelian ratios and showed no differences in body length or weight at birth compared with their littermates, they displayed progressive delays in limb length and body weight gain. We calculated the weight and length of the TSC1KO mice at 4 months, and found that the length was significantly decreased compared with control mice, and body weight was also markedly reduced in the TSC1KO group (Figure 2A). The femora and tibiae of TSC1KO mice were much shorter than those of control mice (Figure 2B,C). These results demonstrate that loss of TSC1 limits limb development and causes dwarfism in mice.
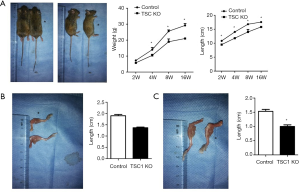
Deletion of TSC1 in limb bud mesenchymal cells causes chondrodysplasia in mice
We next performed histological analysis of limbs of TSC1KO mice. H&E staining showed that TSC1KO mice had significantly thicker growth plates compared to their littermate control mice. This change resulted from the expansion of chondrocytes at all stages in TSC1KO mice, which was most evident in hypertrophic chondrocytes. Furthermore, the size of TSC1-deficient chondrocytes in the hypertrophic chondrocyte zone of the growth plates was also increased significantly (Figure 3A). Measurement of the hypertrophic zone in TSC1KO mice showed that it was 20% shorter than that of control littermates (Figure 3B). These results indicate that deletion of TSC1 in limb bud mesenchyme causes expansion of chondrocytes and of the growth plate, resulting in chondrodysplasia in mice.
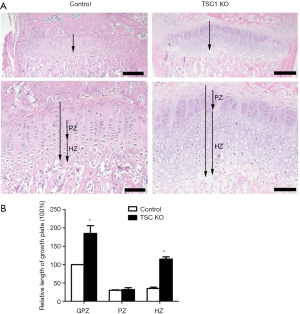
Deletion of TSC1 in limb bud mesenchyme cells causes severe knee joint OA in mice
Ablation of TSC1 in limb bud mesenchyme also induced hyper-activation of mTORC1 in articular cartilage chondrocytes of TSC1KO mice (Figure 1B). To further investigate the effect of limb mesenchyme TSC1 deletion on articular cartilage and joint development, we performed fast green/safranin O staining on sections of knee joint from 8-week-old TSC1KO and control mice. Interestingly, TSC1KO mice developed severe knee joint OA, characterized by loss of proteoglycan from the articular surface and disorganization of chondrocytes. As expected, the Osteoarthritis Research Society International (OARSI) score dramatically increased in TSC1KO mice (Figure 4A). Toluidine blue staining further confirmed severe cartilage erosion and loss of proteoglycans in the articular cartilage of TSC1KO mice (Figure 4B). Taken together, these findings indicated that TSC1-deficiency and mTORC1 activation in limb mesenchyme induce spontaneous and severe knee joint OA in mice.
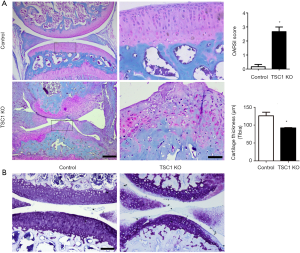
mTORC1 activation in limb bud mesenchymal cells increases subchondral bone mass in mice
Although OA has long been viewed as a primary disorder of articular cartilage, subchondral bone is commonly reported to play a vital role in the pathogenesis of OA (16-18). As TSC1KO mice demonstrated a hyperactivation of mTORC1 in osteoblasts (Figure 1C), we next investigated the effect of limb bud mesenchyme TSC1 disruption on subchondral bone formation. Micro-CT analysis of the femur showed a dramatic increase in subchondral bone mass in 8-week-old TSC1KO mice, as demonstrated by a significant increase in BMD, BV/TV, trabecular number or trabecular thickness, coupled with a decrease in trabecular separation compared with CONTROL mice (Figure 5A,B,C,D,E,F). The volume of subchondral bone was significantly increased in these mutant mice (Figure 5G). IHC staining of subchondral bone displayed a dramatic increase in osteocalcin expression in TSC1KO mice compared with that of the littermate controls (Figure 5H). Taken together, these results demonstrate that TSC1 deletion in limb mesenchymal cells stimulates osteoblastic bone formation and increases subchondral bone mass.
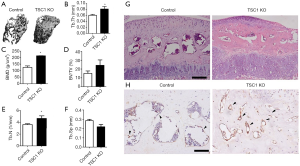
Discussion
The appendicular skeleton of mammals is derived from cartilage templates through endochondral ossification (19). The process begins with condensation of mesenchymal cells within the embryonic limb bud. Subsequently, cells at the core of the mesenchymal condensation differentiate into chondrocytes, which secrete cartilage matrix rich in type II collagen and proteoglycans. Endochondral bone formation depends on a highly coordinated program of proliferation, differentiation and maturation through permanent withdrawal from the cell cycle, hypertrophy and terminal differentiation of chondrocytes within the mammalian growth plate (20-22). Disruption of this program results in chondrodysplasia or a malformed skeleton (21). However, relatively little is known about the intracellular signaling mechanisms responsible for the coordination and transitions involved.
We have recently demonstrated that normal and precisely-controlled mTORC1 activity is essential to coordinate chondrocyte growth, proliferation and differentiation during endochondral bone formation. Hyper-activation of mTORC1 via Tsc1 gene deletion in chondrocytes (Col2a1-Cre) causes uncoupling of the normal proliferation and differentiation program within the growth plate, resulting in uncontrolled cell proliferation, blockage of differentiation, and chondrodysplasia in mice (14). Another study by Chen et al. showed that inactivation of mTORC1 through deletion of either mTOR or the associated protein raptor diminishes embryonic skeletal growth due to a reduction in chondrocyte cell size and the amount of cartilage matrix, resulting in a subsequent severe delay in chondrocyte hypertrophy and bone formation (10). However, the role of TSC1 in limb bud mesenchymal cells and limb development is unclear. Prx1 is one of the earliest genes that are expressed in the mesenchyme of the prospective limb-forming region in mouse embryos (23). We therefore deleted the Tsc1 gene with Prx1-Cre, which targets mainly the limb, the cranial and the interlimb flanking mesenchyme (23). We found that deletion of TSC1 in limb mesenchymal cells causes expansion of chondrocytes and the growth plate, resulting in chondrodysplasia and dwarfism in the knockout mice. Thus, our data suggest that limb bud mesenchymal TSC1 is essential for normal limb development.
The other critical phenotype of the TSC1KO mice is spontaneous and severe knee joint OA. Several recent studies have demonstrated that mTORC1 may play a vital role in chondrocyte metabolism and OA pathophysiology (24). Both cartilage-specific deletion of mTOR and pharmacological inhibition of mTORC1 have been shown to reduce the severity of experimental OA in a mouse model (25,26). We recently showed that mice with ablation of Tsc1 in chondrocytes (col2a1-Cre) also exhibited spontaneous OA, with aberrant chondrocyte proliferation and hypertrophic differentiation (27). This was partially associated with dramatic downregulation of fibroblast growth factor receptor 3 (FGFR3) and parathyroid hormone (PTH)/PTH-related protein (PTHrP) receptor (PPR), two receptors critical for preventing chondrocyte proliferation and differentiation (27). Interestingly, the severity of knee joint OA in Tsc1-Prx1-Cre mice is far more serious than that in Tsc1-col2a1-Cre mice (Figure 4) (27). This difference may result from ablation of TSC1 in cells at an earlier stage (mesenchymal stem cells), since Tsc1-Prx1-Cre interferes with normal knee joint development and causes disorganization of articular cartilage chondrocytes and malformation of the knee joints, while deletion of TSC1 in osteoblasts Tsc1-Prx1-Cre stimulates aberrant osteoblastic bone formation and induces disorganization of the subchondral bone and increased bone mass.
In summary, this study demonstrates that deletion of TSC1 and activation of mTORC1 in limb bud mesenchyme causes chondrodysplasia and severe knee joint OA in mice, implying that mesenchymal TSC1 is essential for normal limb and joint development and function.
Acknowledgments
Funding: This work was supported by project supported by the Natural Science Foundation of Guangdong Province (No. 2018A0303130189, 2017A030310269, 2017A030310438) and the National Natural Science Foundation of China (No. 81800781).
Footnote
Reporting Checklist: The authors have completed the ARRIVE reporting checklist. Available at http://dx.doi.org/10.21037/aoj-17-21
Data Sharing Statement: Available at: http://dx.doi.org/10.21037/aoj-17-21
Conflicts of Interest: All authors have completed the ICMJE uniform disclosure form (available at http://dx.doi.org/10.21037/aoj-17-21). The authors have no conflicts of interest to declare.
Ethical Statement: The authors are accountable for all aspects of the work in ensuring that questions related to the accuracy or integrity of any part of the work are appropriately investigated and resolved. All experiments were performed in accordance and approved by the Southern Medical University Experimental Animal Welfare and Ethics Committee (No. K2018018).
Open Access Statement: This is an Open Access article distributed in accordance with the Creative Commons Attribution-NonCommercial-NoDerivs 4.0 International License (CC BY-NC-ND 4.0), which permits the non-commercial replication and distribution of the article with the strict proviso that no changes or edits are made and the original work is properly cited (including links to both the formal publication through the relevant DOI and the license). See: https://creativecommons.org/licenses/by-nc-nd/4.0/.
References
- Bai X, Jiang Y. Key factors in mTOR regulation. Cell Mol Life Sci 2010;67:239-53. [Crossref] [PubMed]
- Laplante M, Sabatini DM. mTOR signaling in growth control and disease. Cell 2012;149:274-93. [Crossref] [PubMed]
- Shimobayashi M, Hall MN. Making new contacts: the mTOR network in metabolism and signalling crosstalk. Nat Rev Mol Cell Biol 2014;15:155-62. [Crossref] [PubMed]
- Inoki K, Corradetti MN, Guan KL. Dysregulation of the TSC-mTOR pathway in human disease. Nat Genet 2005;37:19-24. [Crossref] [PubMed]
- Barron RP, Kainulainen VT, Forrest CR, et al. Tuberous sclerosis: clinicopathologic features and review of the literature. J Craniomaxillofac Surg 2002;30:361-6. [Crossref] [PubMed]
- Bernauer TA, Mirowski GW, Caldemeyer KS. Tuberous sclerosis. Part II. Musculoskeletal and visceral findings. J Am Acad Dermatol 2001;45:450-2. [Crossref] [PubMed]
- Pui MH, Kong HL, Choo HF. Bone changes in tuberous sclerosis mimicking metastases. Australas Radiol 1996;40:77-9. [Crossref] [PubMed]
- Dickerson WW. Nature of certain osseous lesions in tuberous sclerosis. AMA Arch Neurol Psychiatry 1955;73:525-9. [Crossref] [PubMed]
- Holt JF, Dickerson WW. The osseous lesions of tuberous sclerosis. Radiology 1952;58:1-8. [Crossref] [PubMed]
- Chen J, Long F. mTORC1 signaling controls mammalian skeletal growth through stimulation of protein synthesis. Development 2014;141:2848-54. [Crossref] [PubMed]
- Karner CM, Lee SY, Long F. Bmp Induces Osteoblast Differentiation through both Smad4 and mTORC1 Signaling. Mol Cell Biol 2017;37:e00253-16. [Crossref] [PubMed]
- Huang B, Wang Y, Wang W, et al. mTORC1 Prevents Preosteoblast Differentiation through the Notch Signaling Pathway. PLoS Genet 2015;11:e1005426 [Crossref] [PubMed]
- Riddle RC, Frey JL, Tomlinson RE, et al. Tsc2 is a molecular checkpoint controlling osteoblast development and glucose homeostasis. Mol Cell Biol 2014;34:1850-62. [Crossref] [PubMed]
- Yan B, Zhang Z, Jin D, et al. mTORC1 regulates PTHrP to coordinate chondrocyte growth, proliferation and differentiation. Nat Commun 2016;7:11151. [Crossref] [PubMed]
- Huang B, Wang W, Li Q, et al. Osteoblasts secrete Cxcl9 to regulate angiogenesis in bone. Nat Commun 2016;7:13885. [Crossref] [PubMed]
- Funck-Brentano T, Cohen-Solal M. Subchondral bone and osteoarthritis. Curr Opin Rheumatol 2015;27:420-6. [Crossref] [PubMed]
- Li G, Yin J, Gao J, et al. Subchondral bone in osteoarthritis: insight into risk factors and microstructural changes. Arthritis Res Ther 2013;15:223. [Crossref] [PubMed]
- Hügle T, Geurts J. What drives osteoarthritis?-synovial versus subchondral bone pathology. Rheumatology (Oxford) 2017;56:1461-71. [PubMed]
- Kronenberg HM. Developmental regulation of the growth plate. Nature 2003;423:332-6. [Crossref] [PubMed]
- Long F, Ornitz DM. Development of the endochondral skeleton. Cold Spring Harb Perspect Biol 2013;5:a008334 [Crossref] [PubMed]
- Karaplis AC, Luz A, Glowacki J, et al. Lethal skeletal dysplasia from targeted disruption of theparathyroid hormone-related peptide gene. Genes Dev 1994;8:277-89. [Crossref] [PubMed]
- Wuelling M, Vortkamp A. Chondrocyte proliferation and differentiation. Endocr Dev 2011;21:1-11. [Crossref] [PubMed]
- Logan M, Martin JF, Nagy A, et al. Expression of Cre Recombinase in the developing mouse limb bud driven by a Prxl enhancer. Genesis 2002;33:77-80. [Crossref] [PubMed]
- Pal B, Endisha H, Zhang Y, et al. mTOR: a potential therapeutic target in osteoarthritis? Drugs R D 2015;15:27-36. [Crossref] [PubMed]
- Zhang Y, Vasheghani F, Li YH, et al. Cartilage-specific deletion of mTOR upregulates autophagy and protects mice from osteoarthritis. Ann Rheum Dis 2015;74:1432-40. [Crossref] [PubMed]
- Matsuzaki T, Matsushita T, Tabata Y, et al. Intra-articular administration of gelatin hydrogels incorporating rapamycin-micelles reduces the development of experimental osteoarthritis in a murine model. Biomaterials 2014;35:9904-11. [Crossref] [PubMed]
- Zhang H, Wang H, Zeng C, et al. mTORC1 activation downregulates FGFR3 and PTH/PTHrP receptor in articular chondrocytes to initiate osteoarthritis. Osteoarthritis Cartilage 2017;25:952-63. [Crossref] [PubMed]
Cite this article as: Lai PL, Lu JS, Bai XC, Cai DZ. Deletion of tuberous sclerosis complex 1 (TSC1) in limb bud mesenchyme causes chondrodysplasia and severe knee joint osteoarthritis in mice. Ann Joint 2020;5:39.