Nonsurgical treatments for post-traumatic elbow contractures: approaches for the prevention of their development and progression
Introduction
Contractures is a term used for the loss of motion at a joint. Contractures limit function by interfering with the positioning of the hand in space or with the smooth and efficient progression of gait (1-4). Contractures can be considered based on the etiology and anatomic structure(s) involved. Conditions such as soft-tissue and/or osseous injury, osteoarthritis, inflammatory arthritis, acquired or developmental neurologic deficits and inherited diseases can lead to alterations in periarticular tissues including cartilage, bone, joint capsule, ligaments, muscle/tendons, and skin that lead to contractures (2,5). Post-traumatic contractures have been a recognized complication of fractures and soft-tissue injuries for centuries and remains a relevant concern for patients and practitioners today (2,6). Such contractures are an optimal group to further the understanding of the pathophysiology and management of the condition since the inciting source and time of application/initiation are easier to define when compared to other types of contractures.
The elbow is accepted as a joint prone to developing contractures following injury (4,5,7,8). Reports on various types of elbow fractures and/or dislocations in the literature indicate that 10–15% of the injuries ultimately have operative contracture releases (7,9-11). Operative contracture releases improve motion, but infrequently achieve normal motion (4,5,8,12,13). Given that post-traumatic elbow contractures are a common occurrence with current modern management and that operative releases do not restore normal joint motion, nonsurgical approaches are being explored to prevent the development and progression of, or as adjuvants to surgery for established post-traumatic elbow contractures.
Over the last 60 years investigators have pondered the underlying pathophysiology of post-traumatic contracture development during the healing process after injury (Figure 1). Studies have focused on fibrosis and the resulting collagen alterations in terms of amount and cross-linking (14-18). Other matrix molecules such as decorin, hyaluronic acid, matrix metalloproteinases and their inhibitors, and profibrotic growth factors including transforming growth factor-beta 1 (TGF-β1) and connective tissue growth factor (CTGF) have been examined (16-18). The role of fibroblasts, and their transformation to myofibroblasts has been recognized as a key component to the development of contractures (19-24). Inflammation is also acknowledged as an essential process in the initiation of contracture development (25). Abnormal processes in this phase of healing and the subsequent transition to matrix deposition may set the stage for the aberrant fibrotic events leading to a contracture (25).
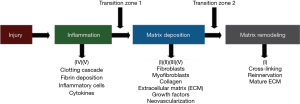
With this background, a summary of the nonsurgical methods is organized via strategies to modify what is known regarding the pathophysiology of post-traumatic contractures. While progress is being made, many issues remain to be resolved, and thus approaches for future work will address platforms, collaborations and strategies to yield an enhanced understanding of the pathophysiologic process.
Nonsurgical interventions
A literature review using MEDLINE (OVID) was conducted with the assistance of a librarian (Supplementary file). Three concepts were developed: anatomy or location; contracture and other words for fibrosis or adhesions; and drug therapy, therapeutic use coupled with specific drugs, biologics, and classes of biologics/drugs. One reviewer (KAH) selected citations where the main topic was post-traumatic contractures, adhesions following immobilization, and nonsurgical interventions.
Most reports from this search of the literature for nonsurgical interventions have been in vivo studies in rats and rabbits. With one exception where the ankle joint was the model, the knee has been the choice of the investigators. Arthrofibrosis is common following elbow and knee injuries and while loading and biologic environments could have unique influences in these joints, following a major injury fibrosis likely has elements common to both joints (4,26). These preclinical models employ combinations of immobilization and/or intra-articular injury to produce contractures and/or adhesions. The immobilization may be internal wires, external casts or splints. The injuries include femoral condyle decortication, periarticular fractures, ligament injuries or capsular disruption. The interventions have been administered in various ways; intra-articular, subcutaneous, intra-muscular or intra-peritoneal injections, or oral ingestion. Single or multiple discrete applications, or continuous administration over extended periods through sustained release pellets or osmotic infusion pumps have been described. In most instances the intervention started at the time of contracture induction although in a few experiments the intervention initiation was delayed for a few weeks. Evaluations have occurred at a few to several weeks after immobilization and/or injury. The outcomes frequently include biomechanical measures of joint ROM and/or stiffness, histology, or mRNA and protein levels of fibrosis markers. With a few exceptions, the relative effectiveness of the various interventions is unable to be determined due to the differing models, outcome measures and single interventions studied. The interventions will be considered based on the rationale that the authors gave for impacting the pathophysiology discussed in the Introduction-collagen modification, fibroblast and myofibroblast anti-proliferatives, hyaluronic acid replacement, anti-inflammatory, growth factors or diuretics (Figure 1).
Collagen (Table 1)
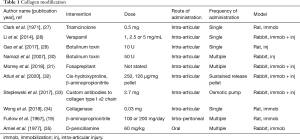
Full table
Collagen is the major protein in periarticular tissues and properties such as amounts and intra- and intermolecular cross-links are potential targets. The amount of collagen can be modified by decreasing production (triamcinolone, verapamil, botulinum toxin A, fosaprepitant, cis-hydroxyproline), interfering with collagen fibril assembly (antibodies to collagen type I α2 chain) or degradation (bacterial collagenase). Collagen cross-links could also be inhibited using β-aminoproprionitrile and D-penicillamine.
A high dose of triamcinolone was reported to decrease joint stiffness, although the authors could not rule out systemic absorption of the intra-articular dose as part of the treatment effect (27). Verapamil is a calcium channel blocker that has effects on myofibroblast collagen synthesis (28). Histologically, adhesion formation was decreased, and within the adhesions the number of fibroblasts and the hydroxyproline content were decreased in a dose-dependent relationship. For the botulinum toxin A experiments, adhesion scores and histologic counts of fibroblasts were decreased (29,30). Knee ROM was improved in association with decreased synovial fluid interleukin-1 and fibroblast growth factor (FGF) levels (29). Fosaprepitant, a neurokinin-1 (NK1) receptor antagonist that limits collagen production by fibroblasts, modified profibrotic genes in the joint capsule (31). However, this was not associated with a decrease in contracture angles. Cis-hydroxyproline was used as a method to interfere with collagen production on the premise that it competes with L-proline, although the investigators found no effect on joint stiffness in the specific conditions tested (32).
Collagen fibril assembly inhibition with antibodies to collagen type I α2 chain delivered continuously via osmotic pump and catheter decreased flexion contractures, supporting the concept of targeting extracellular processes associated with fibrotic changes in periarticular tissues (33). Collagenase (Clostridium histolyticum) was administered when the immobilization device was removed, and after 2 weeks of remobilization, knee extension under a standardized torque was improved in association with increased levels of collagen type III in the posterior joint capsule (34). Conflicting reports on inhibition of collagen cross-links with β-aminoproprionitrile showed joint stiffness significantly reduced (19) or unaffected (32). The difference in dose and/or administering the treatment immediately (19) instead of 8 weeks after injury (32) may explain the discrepancy between studies, although other factors could contribute. D-penicillamine was used successfully to decrease reducible intermolecular Schiff base collagen cross-links in periarticular tissues (35). However, biomechanical measures of joint stiffness were not reported.
These studies illustrate that targeting extracellular and intracellular processes around collagen production, assembly, degradation and cross-linking can modify collagen, other fibrotic molecules, adhesions and fibroblast numbers. However, the biomechanical outcomes were not reported (three interventions), showed no improvement in contractures (three interventions) or improvement in contractures (five interventions).
Anti-proliferatives (Table 2)
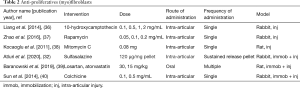
Full table
A number of anti-proliferative compounds have been used for their inhibition of fibroblasts and myofibroblasts, the effector cells in periarticular tissues associated with post-traumatic contractures. The chemotherapeutic drugs 10-hydroxycamptothecin and mitomycin C, and the transplant antirejection drug rapamycin, were shown to decrease adhesion formation (36-38) in association with decreased hydroxyproline content and fibroblast density (36,37). Sulfasalazine was selected for its ability to induce myofibroblast apoptosis (32). Joint stiffness was decreased and histologic analysis reported decreased synovial intimal thickness and α-smooth muscle actin expression (myofibroblast marker) in the joint capsule (32). Authors cited literature indicating the antihypertensive losartan and lipid lowering agent atorvastatin inhibit myofibroblast differentiation and fibroblast proliferation in vitro (39). In their in vivo study there were decreased myofibroblast numbers in the joint capsule, but there was no detectable effect on ROM using either drug (39). Colchicine is a compound that binds the microtubular proteins and disrupts the cytoskeleton of leukocytes and fibroblasts. With colchicine treatment, adhesion scores, hydroxyproline content, and fibroblast numbers were significantly less than controls (40).
The anti-proliferatives appear to decrease adhesions, hydroxyproline content and fibroblasts/myofibroblast numbers. Biomechanical effects of these approaches are uncertain since four of the medications had no such outcome measures performed (36-38,40), and only one of the other three medications decreased joint stiffness even though there were decreases in myofibroblasts (32,39).
Hyaluronic acid (Table 3)
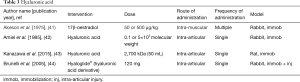
Full table
Hyaluronic acid is a polysaccharide component of the matrix of periarticular issues and could play a role in contractures in many ways. From a biologic perspective, it attracts water and could modify the interactions of the collagen fibers (16,41) and thus, the biomechanical properties of the system. Early studies on immobilized joints noted that hyaluronic acid levels were diminished in the periarticular tissues (16). To counteract this abnormality, strategies tested were the direct administration of hyaluronic acid, or 17β-oestradiol which enhances hyaluronic acid content (41-43). Injections of hyaluronic acid decreased joint stiffness by 50% in concert with increased glycosaminoglycans in the periarticular tissues (42), and mRNA levels for inflammatory, fibrotic and hypoxic molecules were decreased in association with increased posterior joint capsule elasticity on ultrasound (43). Systemic administration of 17β-oestradiol also decreased joint stiffness by 50% with increased glycosaminoglycans in the periarticular tissues of male and female rabbits (41). Hyaloglide® is a hyaluronic acid derivative produced by autocross-linking that was used as a barrier to successfully decrease intra-articular adhesions (44). However, there were no biomechanical, molecular or immunohistochemical measures reported (44).
Hyaluronic acid treatment had beneficial effects on decreasing contracture severity and increasing joint capsule elasticity coupled with enhanced glycosaminoglycans and deceased inflammatory, fibrotic and hypoxic molecules (44). The molecule could act as a physical barrier depending on the viscoelastic properties of the formulation applied. In addition, hyaluronic acid can influence cell activity by binding to CD44 or RHAMM receptors on the surface of cells (45). This multiplicity of functions is an important consideration for future possible applications.
Anti-inflammatory (Table 4)
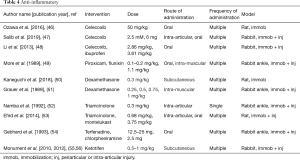
Full table
Since inflammation is acknowledged to play a role in the initiation of post-traumatic contractures, several studies using different classes of medications have been evaluated. Nonsteroidal anti-inflammatory drugs (NSAIDs) and glucocorticoids have been used the most, with additional reports on leukotriene inhibitors, antihistamines and mast cell stabilizers.
Celecoxib is a NSAID that is a cyclooxygenase type 2 enzyme inhibitor. Three different groups reported on the use of celecoxib to prevent contractures and adhesions (46-48). In all three studies, ROM was improved, with one group further characterizing that the improvement was in the myogenic component of the contracture (46), while another group noted improvement in the capsular component (47). The joint capsule mRNA levels for collagen types 1, 3 and 6 were decreased (47). While ibuprofen also improved joint motion when compared to control values, celecoxib had a significantly greater improvement when compared to ibuprofen (48). The nonspecific cyclooxygenase inhibitors piroxicam and flunixin significantly reduced limb swelling, but neither drug improved joint stiffness (49).
Dexamethasone and triamcinolone were the glucocorticoids tested for the prevention of post-traumatic contractures based on their anti-inflammatory properties. Systemic administration of dexamethasone increased joint motion (50,51), while proinflammatory cytokine mRNA levels and fibrotic reactions in the joint capsule were decreased (50). Triamcinolone was injected intra-articularly and biomechanical measures showed that joint motion increased, and joint stiffness decreased (52,53).
Leukotrienes and histamines have been recognized as part of the inflammatory response. The leukotriene inhibitor montelukast significantly improved joint motion (53). Two different antihistamines, terfenadine and chlorpheniramine that were dispensed systemically each significantly reduced joint stiffness (54). Ketotifen was evaluated since it is a mast cell stabilizer preventing the release of cytokines and growth factors (25,55-57). It is also an antihistamine. Systemic administration of ketotifen decreased contracture severity by 50% with concomitant decreases in the number of myofibroblasts and mast cells, and protein and mRNA levels for collagen type I, α-SMA and TGF-β1 in the posterior rabbit knee joint capsule (55,56). Tryptase is a proteinase almost exclusively found in mast cells (58). Serum mast cell tryptase was measured in samples obtained from the rabbits and the levels were reduced 50% in the serum of rabbits receiving ketotifen when compared to control rabbits having the injury and operation, but no medication (57).
Several different anti-inflammatory drug classes have consistently decreased contracture severity with evidence of decreases in fibrotic matrix molecules and cytokines. Specific cyclooxygenase type 2 inhibitors appear to be more effective than nonspecific cyclooxygenase inhibitors. Many of these agents are approved for human use and thus, are worthy of further investigation.
Growth factors (Table 5)
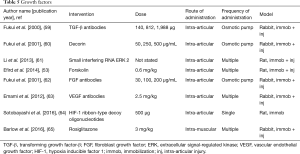
Full table
As inflammation is noted to play an important role in the pathogenesis of post-traumatic joint contractures, several investigators have taken the tactic of modifying key molecules in the inflammatory process. Profibrotic growth factors such as TGF-β1 and CTGF have been the most common targets. Direct interference with the growth factors or upstream and downstream pathways of the growth factors have been reported.
In separate experiments, investigators directly inhibited TGF-β1 using neutralizing antibodies or decorin, which binds TGF-β1 in the matrix, delivered via an osmotic pump and catheter (59,60). In both studies, adhesion scores, biomechanical measures of stiffness and severity of joint motion loss, and collagen content in adhesion tissue were decreased.
Three studies by different authors modified pathways up or downstream from TGF-β1. Extracellular signal-regulated kinase (ERK) 1/2 is a downstream effector molecule facilitating fibroblast proliferation and collagen synthesis, and phosphorylated ERK2 levels are increased in post-traumatic joint contractures (61). Small interfering RNA that directs the degradation of targeted RNA (ERK2) was manufactured and injected via a lentivirus vector (61). Flexion contractures were significantly decreased in association with decreased protein levels of phosphorylated ERK2 and total collagen, and myofibroblast numbers in the joint capsule. Another group investigated rosiglitazone, a peroxisome proliferator-activated receptor-gamma agonist which modulates TGF-β1 as an anti-fibrotic agent (65). Systemic administration of rosiglitazone demonstrated that 10 genes and 17 pathways associated with fibrosis were significantly modified in the joint capsule. However, joint ROM and myofibroblast numbers were not improved (65). The final method reported to modulate TGF-β1 is the application of forskolin, an agent that stimulates cyclic adenosine monophosphate (c-AMP). The authors postulated that proinflammatory cytokine production, including TGF-β1, would decrease through activation of protein kinase A via the elevated c-AMP induced by forskolin (53). Biomechanical tests showed that knee joint extension was significantly improved in the forskolin group.
Three different investigations targeted FGF, CTGF or vascular endothelial growth factor (VEGF). Neutralizing antibodies to FGF-2 delivered via an osmotic pump and catheter decreased contracture severity and adhesion formation with increasing doses of antibodies (62). In another study, investigators used an antibody to VEGF and determined that contracture severity and macroscopic adhesion grading were significantly reduced (63). Fibroblast numbers, vascularity and collagen matrix deposition were also decreased. The third study focused on hypoxia inducible factor 1 (HIF-1), a transcription factor that upregulates VEGF and CTGF expression (64). Ribbon decoy oligodeoxynucleotides (ODNs) were constructed that bind to free HIF-1 which resulted in blockade of HIF-1 attachment to the promotor region for VEGF and CTGF. Contracture severity was decreased with the HIF-1 ODN in association with decreased mRNA levels for VEGF and CTGF.
Modifying growth factors decreased contracture severity in seven of eight studies. This was associated with decreased measures of fibrosis and adhesions. The direct inhibition, and upstream and downstream regulation, give the strongest evidence for TGF-β1 involvement. Issues such as delivery method, dose, timing of intervention and having no currently approved TGF-β1 will need to be addressed before human trials can be conducted.
Diuretics (Table 6)

Full table
Swelling and tissue edema are associated with periarticular fractures as is joint stiffness (66). Diuretics are used in hypertension and congestive heart failure for their ability to mobilize edema fluid (67). After a periarticular ankle fracture stabilized with external immobilization the diuretics chlorothiazide and acetazolamide decreased biomechanical measures of joint stiffness with no statistical differences between the diuretics (66). There was no detectable effect on measures of joint swelling with the diuretics, and thus the mechanism through which the diuretics modified joint stiffness is unknown.
Human elbow studies (Table 7)
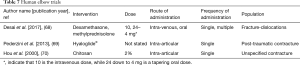
Full table
Three studies were identified where nonsurgical interventions were used as adjuncts to improve joint motion following operative procedures on the elbow. The population was acute trauma (68), post-traumatic contractures (69), or unspecified elbow contracture (70).
In a retrospective study of terrible triad elbow fracture-dislocations managed with operative stabilization of soft-tissues, coronoid fracture fixation and radial head arthroplasty, 13 participants received an intra-operative dexamethasone dose of 10 mg intra-venously followed by a 6-day tapering dose of oral methylprednisolone from 24 to 4 mg while an additional 13 participants did not receive any glucocorticoids (68). The elbows were immobilized for 2 weeks before a hand therapist delivered a supervised physiotherapy program. Follow up occurred at 2, 6, 12 and 24 weeks where ROM was measured with a handheld goniometer. It was determined that there was a significantly greater ROM in the flexion-extension arc of elbow motion at 24 weeks with glucocorticoid treatment.
The other two studies investigated results after operative release in a population with established elbow stiffness. An arthroscopic arthrolysis was performed on 36 participants with post-traumatic elbow contractures (69). Seventeen participants had an intra-articular application of the autocross-linked hyaluronic acid gel Hyaloglide® as a mechanical barrier to adhesion formation and tissue lubricator, while 19 participants had no anti-adhesive treatment. Elbow ROM measurements and the Liverpool Elbow Score (LES) were gathered 30 and 75 days after the operation. The ROM and LES measures improved significantly over time in both groups with no statistically significant differences between the groups. The third study from China has an English abstract only (70). The investigators used chitosan as a single intra-articular injection to prevent adhesions following elbow arthrolysis to manage elbow stiffness. The cause of stiffness was not indicated. There were 12 participants who received the chitosan, while 14 participants did not. Improvement in elbow ROM following surgery occurred in both groups, with a significantly greater increase in the chitosan group an average of 24 months after the operation.
These clinical trials are an important step in identifying effective interventions. The information is limited as none of the studies were randomized clinical trials and the number of participants small, 26 or 36 in each study.
There are 40 interventions included in the 37 studies represented in this review. While a single direction emanating from this review is lacking, the following considerations are noted. Methods dampening inflammation and/or altering growth factors have been the most consistent in limiting contractures. Some of these approaches are more recent and show promise, but there are possibilities of serious adverse events such as negating tumor protective properties of TGF-β1 for example. Older studies modifying collagen properties (cis-hydroxyproline) or myofibroblast numbers (mitomycin C) have unknown or inconsistent effects on contracture severity while having concerns for systemic side effects, which may explain no further recent studies. Mode of delivery is an important factor. Oral administration is less invasive than injections. Local application may be necessary to have an effect and/or limit side effects from systemic administration.
Strategies for clinical innovation
There has been considerable effort examining various nonsurgical interventions using animal models with relatively little translation to clinical settings or developing unifying theories of fibrosis pathogenesis. It is clear that to move forward, a collaborative approach with multiple elements in a platform is required (Figure 2). The platforms serve unique, complimentary and overlapping purposes.
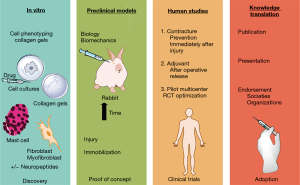
In vitro manipulation of fibroblasts and myofibroblasts obtained from joint capsules from human and animal model sources allows rapid screening of potential interventions and facilitates mechanistic and discovery research directions (24,71-77). Various properties of the fibroblasts have been measured including collagen gel contraction, protein and mRNA synthesis, proliferation, viability and migration. The preclinical in vivo models allow for validation, or proof of concept for interventions identified through in vitro studies. The biomechanical tests of contracture and biologic evaluations are methods to show efficacy, and also can be used to support discovery lines of investigation. Demonstrating that pathways and genes associated with fibrosis in the joint capsule and other periarticular tissues contributing to contractures, and that serum markers, are modified shed light on the mechanisms associated with biomechanical alterations (57,65). The preclinical studies are also necessary to answer questions on dosing, routes and timing of administration.
Randomized clinical trials are the gold standard for establishing efficacy and reporting on the safety of interventions (Figure 2). There are several factors to consider. An intervention already approved for human use that requires repurposing of a medication for a new indication (e.g., NSAIDs) has many fewer hurdles and unknowns compared to an intervention not previously approved for human use. Large multicenter trials are frequently indicated to recruit appropriate numbers of patients. The patient population matters. The effect of sex and age on contracture development is poorly understood but is a recognized contributor to other musculoskeletal conditions such as osteoporosis (26). Injury severity has been implicated as a predisposing factor as represented by having an operation or not in a clinical trial of elbow fractures and dislocations, and in a New York State database review of operative elbow injuries having subsequent operations for contracture release (78,79). Recent work has examined the potential role of serum mast cell tryptase as a biomarker (57,78). Since approximately 15% of elbow injuries ultimately have operative procedures for post-traumatic contracture releases, defining the populations at risk for post-traumatic contractures will advance understanding of the underlying processes, enhance the likelihood of clinical trials identifying a treatment effect, and address the risk-benefit ratio of these nonsurgical interventions in appropriate populations.
While clinical trials provide the necessary information on efficacy and safety for interventions, knowledge translation strategies are essential to facilitate the adoption of the interventions into practice. The scientific approach to presenting results at meetings and to publish journal articles is an important step for peer-review. However, engagement of specialty societies and organizations that serve health care providers and recipients (patients) increase the likelihood that interventions will have widespread adoption in clinical practice (Figure 2).
An example of this approach is the use of ketotifen for the prevention of post-traumatic joint contractures. Investigations of the changes in the joint capsule in human elbows and a validated rabbit knee model of post-traumatic contractures detected increased numbers of myofibroblasts, mast cells, and nerve fibers containing neuropeptides (20,21,80). A theory for the pathogenesis of the capsular fibrosis was based on a myofibroblast-mast cell-neuropeptide fibrosis network (Figure 3). In this scheme, the fibroblast and myofibroblast are the effector cells with the machinery to produce collagen and the contractile capacity to manipulate the collagen, thus producing a shortened and less compliant matrix. With its store of cytokines and bioactive molecules (e.g., TGF-β1), the mast cell could be a significant contributor to the increased collagen production and the transformation of fibroblasts to the more contractile myofibroblasts. The neuropeptide containing nerve fibers in the joint capsule can release substance P in a reflex response to pain and tissue injury with a fracture, which then can interact with neuropeptide receptors (NK1) on the mast cell (81,82).
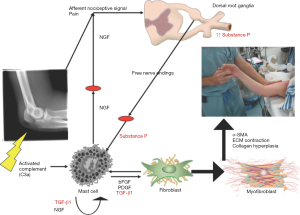
Using the in vitro method of collagen gel contraction assays for discovery research to evaluate the fibrosis network and to screen ketotifen as a suitable candidate for preclinical trials (Figure 2), myofibroblasts from capsules of elbow joints with post-traumatic contractures were tested alone or in combination with human mast cells (71). Agents enhancing (substance P) or inhibiting (ketotifen) mast cell degranulation enhanced or decreased collagen gel contraction of myofibroblasts, respectively. These agents had no direct effect on the myofibroblasts’ ability to contract collagen gels, and mast cells had to be present before these agents affected collagen gel contraction. These findings support the interplay of myofibroblasts, mast cells and neuropeptides in post-traumatic contractures (discovery) and that the mast cell stabilizer ketotifen is a candidate intervention to prevent post-traumatic contractures (directing in vivo studies). Ketotifen was selected for in vivo studies as it is an oral medication that has been used for over 40 years in the treatment of asthma and it is safe (83-85). Positive results could see the translation to a clinical setting quickly. Using a validated preclinical rabbit knee model, proof of concept (Figure 2) for ketotifen to prevent post-traumatic contractures was achieved. Joint contracture severity was significantly reduced by half with concomitant decreases in joint capsule fibrosis and levels of serum mast cell tryptase (55-57). Interestingly from a discovery perspective, the interplay between myofibroblasts, mast cells and neuropeptide is complex in vivo as measures for all three components were decreased in the joint capsule in association with ketotifen treatment suggesting feedback loops amongst these components. These investigations have been followed by a pilot clinical trial (ClinicalTrials.gov NCT01902017), that has led to a multicenter randomized clinical trial (NCT03582176) evaluating ketotifen for safety and ultimately efficacy (86). The second trial is actively recruiting, and it is too early for the knowledge translation and adoption to clinical practice phases of the platform (Figure 2).
Conclusions
Several in vivo studies using animal models of post-traumatic contractures and intra-articular adhesion formation have targeted inflammation, growth factors, fibroblasts and myofibroblasts, collagen, and hyaluronic acid. There are only three clinical trials published, all of which are small and not randomized. An integrated approach is recommended using a platform of in vitro studies for discovery and to screen potential nonsurgical interventions; in vivo preclinical studies for proof of concept and to validate discovery aimed at pathophysiology; well designed, large randomized clinical trials for efficacy and safety; and finally knowledge translation methods to increase the likelihood that efficacious interventions are adopted into clinical practice. Molecular biologists, bioengineers, clinicians, clinical trialists, biostatisticians, and knowledge translation practitioners/organizations will all be key stakeholders. Insights gained on understanding post-traumatic contractures will likely also be relevant to other musculoskeletal conditions characterized by joint contractures and other organ systems affected by fibrosis.
Acknowledgments
The authors thank Kelsey Collins, PhD for assistance with Figures 1,2.
Funding: None.
Footnote
Provenance and Peer Review: This article was commissioned by the Guest Editor (Spencer P. Lake) for the series “Emerging Trends in Elbow Injury, Pathology and Treatment” published in Annals of Joint. The article has undergone external peer review.
Conflicts of Interest: All authors have completed the ICMJE uniform disclosure form (available at http://dx.doi.org/10.21037/aoj-20-62). The series “Emerging Trends in Elbow Injury, Pathology and Treatment” was commissioned by the editorial office without any funding or sponsorship. The authors have no other conflicts of interest to declare.
Ethical Statement: The authors are accountable for all aspects of the work in ensuring that questions related to the accuracy or integrity of any part of the work are appropriately investigated and resolved.
Open Access Statement: This is an Open Access article distributed in accordance with the Creative Commons Attribution-NonCommercial-NoDerivs 4.0 International License (CC BY-NC-ND 4.0), which permits the non-commercial replication and distribution of the article with the strict proviso that no changes or edits are made and the original work is properly cited (including links to both the formal publication through the relevant DOI and the license). See: https://creativecommons.org/licenses/by-nc-nd/4.0/.
References
- Fergusson D, Hutton B, Drodge A. The epidemiology of major joint contractures: a systematic review of the literature. Clin Orthop 2007.22-9. [Crossref] [PubMed]
- Perry J. Contractures. A historical perspective. Clin Orthop 1987.8-14. [PubMed]
- Morrey BF, Askew LJ, Chao EY. A biomechanical study of normal functional elbow motion. J Bone Joint Surg Am 1981;63:872-7. [Crossref] [PubMed]
- Adolfsson L. Post-traumatic stiff elbow. EFORT Open Rev 2018;3:210-6. [Crossref] [PubMed]
- Charalambous CP, Morrey BF. Posttraumatic elbow stiffness. J Bone Joint Surg Am 2012;94:1428-37. [Crossref] [PubMed]
- Ficke JR, Bosse M. Extremity war injuries V: barriers to return of function and duty. J Am Acad Orthop Surg 2011;19 Suppl 1:v-viii. [Crossref] [PubMed]
- Lindenhovius AL, Jupiter JB. The posttraumatic stiff elbow: a review of the literature. J Hand Surg Am 2007;32:1605-23. [Crossref] [PubMed]
- Mellema JJ, Lindenhovius AL, Jupiter JB. The posttraumatic stiff elbow: an update. Curr Rev Musculoskelet Med 2016;9:190-8. [Crossref] [PubMed]
- Doornberg JN, van Duijn PJ, Linzel D, et al. Surgical treatment of intra-articular fractures of the distal part of the humerus. Functional outcome after twelve to thirty years. J Bone Joint Surg Am 2007;89:1524-32. [Crossref] [PubMed]
- Forthman C, Henket M, Ring DC. Elbow dislocation with intra-articular fracture: the results of operative treatment without repair of the medial collateral ligament. J Hand Surg Am 2007;32:1200-9. [Crossref] [PubMed]
- Myden C, Hildebrand K. Elbow joint contracture after traumatic injury. J Shoulder Elbow Surg 2011;20:39-44. [Crossref] [PubMed]
- Lindenhovius AL, Doornberg JN, Ring D, et al. Health status after open elbow contracture release. J Bone Joint Surg Am 2010;92:2187-95. [Crossref] [PubMed]
- Sharma S, Rymaszewski LA. Open arthrolysis for post-traumatic stiffness of the elbow: results are durable over the medium term. J Bone Joint Surg Br 2007;89:778-81. [Crossref] [PubMed]
- Evans EB, Eggers G, Butler JK, et al. Experimental immobilization and remobilization of rat knee joints. JBJS 1960;42:737-58. [Crossref]
- Akeson WH. An experimental study of joint stiffness. J Bone Joint Surg Am 1961;43-A:1022-34. [Crossref] [PubMed]
- Akeson WH, Amiel D, Woo SL. Immobility effects on synovial joints the pathomechanics of joint contracture. Biorheology 1980;17:95-110. [Crossref] [PubMed]
- Hildebrand KA, Zhang M, Hart DA. Joint capsule matrix turnover in a rabbit model of chronic joint contractures: correlation with human contractures. J Orthop Res 2006;24:1036-43. [Crossref] [PubMed]
- Hildebrand KA, Zhang M, Hart DA. High rate of joint capsule matrix turnover in chronic human elbow contractures. Clin Orthop 2005.228-34. [Crossref] [PubMed]
- Furlow LT Jr, Peacock EE Jr. Effect of beta-aminopropionitrile on joint stiffness in rats. Ann Surg 1967;165:442-7. [Crossref] [PubMed]
- Hildebrand KA, Sutherland C, Zhang M. Rabbit knee model of post-traumatic joint contractures: the long-term natural history of motion loss and myofibroblasts. J Orthop Res 2004;22:313-20. [Crossref] [PubMed]
- Hildebrand KA, Zhang M, van Snellenberg W, et al. Myofibroblast numbers are elevated in human elbow capsules after trauma. Clin Orthop Relat Res 2004.189-97. [Crossref] [PubMed]
- Doornberg JN, Bosse T, Cohen MS, et al. Temporary presence of myofibroblasts in human elbow capsule after trauma. J Bone Joint Surg Am 2014;96:e36. [Crossref] [PubMed]
- Abdel MP, Morrey ME, Barlow JD, et al. Myofibroblast cells are preferentially expressed early in a rabbit model of joint contracture. J Orthop Res 2012;30:713-9. [Crossref] [PubMed]
- Mattyasovszky SG, Wollstadter J, Martin A, et al. Inhibition of contractile function in human joint capsule myofibroblasts by targeting the TGF-beta1 and PDGF pathways. PLoS One 2016;11:e0145948. [Crossref] [PubMed]
- Monument MJ, Hart DA, Salo PT, et al. Posttraumatic elbow contractures: targeting neuroinflammatory fibrogenic mechanisms. J Orthop Sci 2013;18:869-77. [Crossref] [PubMed]
- Usher KM, Zhu S, Mavropalias G, et al. Pathological mechanisms and therapeutic outlooks for arthrofibrosis. Bone Res 2019;7:9. [Crossref] [PubMed]
- Clark DD, Weckesser EC. The influence of triamcinolone acetonide on joint stiffness in the rat. J Bone Joint Surg Am 1971;53:1409-14. [Crossref] [PubMed]
- Li Y, Ma X, Yu P, et al. Intra-articular adhesion reduction after knee surgery in rabbits by calcium channel blockers. Med Sci Monit 2014;20:2466-71. [Crossref] [PubMed]
- Gao ZY, Wu JX, Liu WB, et al. Reduction of adhesion formation after knee surgery in a rat model by botulinum toxin A. Biosci Rep 2017;37:BSR20160460. [Crossref] [PubMed]
- Namazi H, Torabi S. Novel use of botulinum toxin to ameliorate arthrofibrosis: an experimental study in rabbits. Toxicol Pathol 2007;35:715-8. [Crossref] [PubMed]
- Morrey ME, Sanchez-Sotelo J, Lewallen EA, et al. Intra-articular injection of a substance P inhibitor affects gene expression in a joint contracture model. J Cell Biochem 2018;119:1326-36. [Crossref] [PubMed]
- Atluri K, Brouillette MJ, Seol D, et al. Sulfasalazine resolves joint stiffness in a rabbit model of arthrofibrosis. J Orthop Res 2020;38:629-38. [Crossref] [PubMed]
- Steplewski A, Fertala J, Beredjiklian PK, et al. Blocking collagen fibril formation in injured knees reduces flexion contracture in a rabbit model. J Orthop Res 2017;35:1038-46. [Crossref] [PubMed]
- Wong K, Trudel G, Laneuville O. Intra-articular collagenase injection increases range of motion in a rat knee flexion contracture model. Drug Des Devel Ther 2017;12:15-24. [Crossref] [PubMed]
- Amiel D, Akeson WH, Harwood FL, et al. Effect of low dosage schedule of D-penicillamine on collagen cross-linking in a nine week immobilized rabbit knee. Connect Tissue Res 1977;5:179-83. [Crossref] [PubMed]
- Liang Y, Sun Y, Li X, et al. The optimal concentration of topical hydroxycamptothecin in preventing intraarticular scar adhesion. Sci Rep 2014;4:4621. [Crossref] [PubMed]
- Zhao S, Sun Y, Li X, et al. Reduction of intraarticular adhesion of knee by local application of rapamycin in rabbits via inhibition of fibroblast proliferation and collagen synthesis. J Orthop Surg Res 2016;11:45. [Crossref] [PubMed]
- Kocaoglu B, Akgun U, Nalbantoglu U, et al. Adhesion reduction after knee surgery in a rat model by mitomycin C. Knee Surg Sports Traumatol Arthrosc 2011;19:94-8. [Crossref] [PubMed]
- Baranowski A, Schlemmer L, Forster K, et al. Effects of losartan and atorvastatin on the development of early posttraumatic joint stiffness in a rat model. Drug Des Devel Ther 2019;13:2603-18. [Crossref] [PubMed]
- Sun Y, Liang Y, Hu J, et al. Reduction of intraarticular adhesion by topical application of colchicine following knee surgery in rabbits. Sci Rep 2014;4:6405. [Crossref] [PubMed]
- Akeson WH, Woo SL, Amiel D, et al. Value of 17beta-oestradiol in prevention of contracture formation. Ann Rheum Dis 1975;35:429-36. [Crossref] [PubMed]
- Amiel D, Frey C, Woo SL, et al. Value of hyaluronic acid in the prevention of contracture formation. Clin Orthop Relat Res 1985.306-11. [Crossref] [PubMed]
- Kanazawa K, Hagiwara Y, Tsuchiya M, et al. Preventing effects of joint contracture by high molecular weight hyaluronan injections in a rat immobilized knee model. Int J Clin Exp Pathol 2015;8:3426-40. [PubMed]
- Brunelli G, Longinotti C, Bertazzo C, et al. Adhesion reduction after knee surgery in a rabbit model by hyaloglide, a hyaluronan derivative gel. J Orthop Res 2005;23:1377-82. [PubMed]
- Altman R, Bedi A, Manjoo A, et al. Anti-inflammatory effects of intra-articular hyaluronic acid: a systematic review. Cartilage 2019;10:43-52. [Crossref] [PubMed]
- Ozawa J, Kaneguchi A, Tanaka R, et al. Cyclooxygenase-2 inhibitor celecoxib attenuates joint contracture following immobilization in rat knees. BMC Musculoskelet Disord 2016;17:446. [Crossref] [PubMed]
- Salib CG, Reina N, Trousdale WH, et al. Inhibition of COX-2 pathway as a potential prophylaxis against arthrofibrogenesis in a rabbit model of joint contracture. J Orthop Res 2019;37:2609-20. [Crossref] [PubMed]
- Li F, He B, Liu S, et al. Celecoxib effectively inhibits the formation of joint adhesions. Exp Ther Med 2013;6:1507-11. [Crossref] [PubMed]
- More RC, Kody MH, Kabo JM, et al. The effects of two nonsteroidal antiinflammatory drugs on limb swelling, joint stiffness, and bone torsional strength following fracture in a rabbit model. Clin Orthop Relat Res 1989.306-12. [Crossref] [PubMed]
- Kaneguchi A, Ozawa J, Yamaoka K. Anti-inflammatory drug dexamethasone treatment during the remobilization period improves range of motion in a rat knee model of joint contracture. Inflammation 2018;41:1409-23. [Crossref] [PubMed]
- Grauer JD, Kabo JM, Dorey FJ, et al. The effects of dexamethasone on periarticular swelling and joint stiffness following fracture in a rabbit hindlimb model. Clin Orthop Relat Res 1989.277-84. [PubMed]
- Namba RS, Kabo JM, Dorey FJ, et al. Intra-articular corticosteroid reduces joint stiffness after an experimental periarticular fracture. J Hand Surg Am 1992;17:1148-53. [Crossref] [PubMed]
- Efird W, Kellam P, Yeazell S, et al. An evaluation of prophylactic treatments to prevent post traumatic joint stiffness. J Orthop Res 2014;32:1520-4. [Crossref] [PubMed]
- Gebhard JS, Johnston-Jones K, Kody MH, et al. Effects of antihistamines on joint stiffness and bone healing after periarticular fracture. J Hand Surg Am 1993;18:1080-5. [Crossref] [PubMed]
- Monument MJ, Hart DA, Befus AD, et al. The mast cell stabilizer ketotifen fumarate lessens contracture severity and myofibroblast hyperplasia: a study of a rabbit model of posttraumatic joint contractures. J Bone Joint Surg Am 2010;92:1468-77. [Crossref] [PubMed]
- Monument MJ, Hart DA, Befus AD, et al. The mast cell stabilizer ketotifen reduces joint capsule fibrosis in a rabbit model of post-traumatic joint contractures. Inflamm Res 2012;61:285-92. [Crossref] [PubMed]
- Kopka M, Monument MJ, Befus AD, et al. Serum mast cell tryptase as a marker of posttraumatic joint contracture in a rabbit model. J Orthop Trauma 2017;31:e86-9. [Crossref] [PubMed]
- Gruber BL. Mast cells in the pathogenesis of fibrosis. Curr Rheumatol Rep 2003;5:147-53. [Crossref] [PubMed]
- Fukui N, Tashiro T, Hiraoka H, et al. Adhesion formation can be reduced by the suppression of transforming growth factor-beta1 activity. J Orthop Res 2000;18:212-9. [Crossref] [PubMed]
- Fukui N, Fukuda A, Kojima K, et al. Suppression of fibrous adhesion by proteoglycan decorin. J Orthop Res 2001;19:456-62. [Crossref] [PubMed]
- Li F, Liu S, Fan C. Lentivirus-mediated ERK2 siRNA reduces joint capsule fibrosis in a rat model of post-traumatic joint contracture. Int J Mol Sci 2013;14:20833-44. [Crossref] [PubMed]
- Fukui N, Nakajima K, Tashiro T, et al. Neutralization of fibroblast growth factor-2 reduces intraarticular adhesions. Clin Orthop Relat Res 2001.250-8. [Crossref] [PubMed]
- Emami MJ, Jaberi FM, Azarpira N, et al. Prevention of arthrofibrosis by monoclonal antibody against vascular endothelial growth factor: a novel use of bevacizumab in rabbits. Orthop Traumatol Surg Res 2012;98:759-64. [Crossref] [PubMed]
- Sotobayashi D, Kawahata H, Anada N, et al. Therapeutic effect of intra-articular injection of ribbon-type decoy oligonucleotides for hypoxia inducible factor-1 on joint contracture in an immobilized knee animal model. J Gene Med 2016;18:180-92. [Crossref] [PubMed]
- Barlow JD, Morrey ME, Hartzler RU, et al. Effectiveness of rosiglitazone in reducing flexion contracture in a rabbit model of arthrofibrosis with surgical capsular release: a biomechanical, histological, and genetic analysis. Bone Joint Res 2016;5:11-7. [Crossref] [PubMed]
- Safran MR, Kody MH, Kabo JM, et al. The effects of diuretics on posttraumatic joint stiffness and limb swelling in a rabbit periarticular fracture model. Clin Orthop Relat Res 1994.284-9. [Crossref] [PubMed]
- Opie LH. Hypertension, the changing pattern of drug usage. Cardiovasc J Afr 2009;20:52-6. [PubMed]
- Desai MJ, Matson AP, Ruch DS, et al. Perioperative glucocorticoid administration improves elbow motion in terrible triad injuries. J Hand Surg Am 2017;42:41-6. [Crossref] [PubMed]
- Pederzini LA, Milandri L, Tosi M, et al. Preliminary clinical experience with hyaluronan anti-adhesion gel in arthroscopic arthrolysis for posttraumatic elbow stiffness. J Orthop Traumatol 2013;14:109-14. [Crossref] [PubMed]
- Hou C, Chen A, Zhang W. Clinical study on chitosan for prevention of elbow adhesion. Zhongguo Xiu Fu Chong Jian Wai Ke Za Zhi 2000;14:80-2. [PubMed]
- Hildebrand KA, Zhang M, Befus AD, et al. A myofibroblast-mast cell-neuropeptide axis of fibrosis in post-traumatic joint contractures: an in vitro analysis of mechanistic components. J Orthop Res 2014;32:1290-6. [Crossref] [PubMed]
- Mattyasovszky SG, Hofmann A, Brochhausen C, et al. The effect of the pro-inflammatory cytokine tumor necrosis factor-alpha on human joint capsule myofibroblasts. Arthritis Res Ther 2010;12:R4. [Crossref] [PubMed]
- Mattyasovszky SG, Mausbach S, Ritz U, et al. Influence of the anti-inflammatory cytokine interleukin-4 on human joint capsule myofibroblasts. J Orthop Res 2017;35:1290-8. [Crossref] [PubMed]
- Mattyasovszky SG, Mausbach S, Ritz U, et al. Cytokine Interferon-gamma suppresses the function of capsule myofibroblasts and induces cell apoptosis. J Orthop Res 2017;35:2524-33. [Crossref] [PubMed]
- Yu D, Zhuang Z, Ren J, et al. Hyaluronic acid-curcumin conjugate suppresses the fibrotic functions of myofibroblasts from contractive joint by the PTGER2 demethylation. Regen Biomater 2019;6:269-77. [Crossref] [PubMed]
- Zhuang Z, Yu D, Chen Z, et al. Curcumin inhibits joint contracture through PTEN demethylation and targeting PI3K/Akt/mTOR pathway in myofibroblasts from human joint capsule. Evid Based Complement Alternat Med 2019;2019:4301238. [Crossref] [PubMed]
- Trousdale WH, Salib CG, Reina N, et al. A drug eluting scaffold for the treatment of arthrofibrosis. Tissue Eng Part C Methods 2018;24:514-23. [Crossref] [PubMed]
- Liu CS, Ademola A, Zhang M, et al. Human serum mast cell tryptase levels in elbow fractures or dislocations and its association with injury severity. J Orthop Res 2020;38:2015-9. [Crossref] [PubMed]
- Schrumpf MA, Lyman S, Do H, et al. Incidence of postoperative elbow contracture release in New York State. J Hand Surg Am 2013;38:1746-52.e1-3.
- Hildebrand KA, Zhang M, Salo PT, et al. Joint capsule mast cells and neuropeptides are increased within four weeks of injury and remain elevated in chronic stages of posttraumatic contractures. J Orthop Res 2008;26:1313-9. [Crossref] [PubMed]
- Peng L, Agogo GO, Guo J, et al. Substance P and fibrotic diseases. Neuropeptides 2019;76:101941. [Crossref] [PubMed]
- Foreman JC. Substance P and calcitonin gene-related peptide: effects on mast cells and in human skin. Int Arch Allergy Appl Immunol 1987;82:366-71. [Crossref] [PubMed]
- Schwarzer G, Bassler D, Mitra A, et al. Ketotifen alone or as additional medication for long-term control of asthma and wheeze in children. Cochrane Database Syst Rev 2004.CD001384. [PubMed]
- Jeffreys DB, Volans GN. Ketotifen overdose: surveillance of the toxicity of a new drug. Br Med J (Clin Res Ed) 1981;282:1755-6. [Crossref] [PubMed]
- Maclay WP, Crowder D, Spiro S, et al. Postmarketing surveillance: practical experience with ketotifen. Br Med J (Clin Res Ed) 1984;288:911-4. [Crossref] [PubMed]
- Ademola A, Hildebrand KA, Schneider PS, et al. PrEvention of posttraumatic contractuRes with Ketotifen 2 (PERK 2) - protocol for a multicenter randomized clinical trial. BMC Musculoskelet Disord 2020;21:123. [Crossref] [PubMed]
Cite this article as: Hildebrand KA, Ademola A, Hart DA. Nonsurgical treatments for post-traumatic elbow contractures: approaches for the prevention of their development and progression. Ann Joint 2021;6:8.