Hip resurfacing arthroplasty—what has history taught us?
Historical perspective
The concept of hip resurfacing is credited to Smith Peterson with his hemi-resurfacing mould arthroplasty in 1948 (1). A lack of reliable fixation and unpredictable outcomes limited its widespread use. In the 1960s, the Charnley low friction total hip arthroplasty was developed. Its success demonstrated the ability of polymethylmethacrylate cement to achieve durable implant fixation, and the acceptable wear characteristics of a cobalt chromium femoral head articulating with a polyethylene acetabular shell (2). Despite excellent results in older patients, concern over implant longevity limited its use in younger patients (3). Hip resurfacing was re-visited as an attractive alternative in younger patients, with the theoretical advantages of bone conservation and easier, simpler revision.
In the 1970s, the total hip articular replacement using internal eccentric shells (THARIES) was developed based on the success of the CoCr-on-polyethylene Charnley total hip arthroplasty. Its design included an all polyethylene, cemented, acetabular liner and cemented cobalt chrome femoral head (4). Other similar designs of the same era included the Indiana Hip resurfacing and Wagner hip resurfacing (5,6). Outcomes were comparable to those of total hip arthroplasty designs available during the same time period with good short term outcomes but poor long term survivorship, primarily due to polyethylene wear and acetabular component loosening (7,8). The cementless, porous surface replacement (PSR) designs succeeded the THARIES in the 1980s and utilized porous coating or sintered beads for bone fixation on both the acetabular shell with modular polyethylene liner and the cobalt chrome femoral component (9). These designs, while able to achieve adequate initial cementless fixation, failed at a similar rate as the THARIES, but with more failures of the femoral than acetabular component seen due to high wear rate of the thin polyethylene line (10).
By the early 1990s, it was recognized that high rates of polyethylene wear from large femoral heads were responsible for the osteolysis and aseptic loosening of these early designs. At the same time, improvements in small diameter head metal-on-metal total hip arthroplasties had led to newer designs with encouraging early results (11). Building on the knowledge of failed implant designs and careful examination of large head metal-on-metal McKee-Farrar components with good long term survival, McMinn and Wagner both developed metal-on-metal hip resurfacing arthroplasties in the early 1990s and brought hip resurfacing into its modern era (12,13).
In the more than 20 years since the first modern, metal-on-metal hip resurfacing was implanted, its advantages over traditional total hip arthroplasty in young, active patients have been well established. However, concern over complications from metal wear has continued to limit its widespread use. The history of modern hip resurfacing has taught us that implant design, patient selection and surgeon experience are all critical to achieving a successful, long term result.
Advantages over traditional total hip arthroplasty
Surface replacement arthroplasty has several potential benefits over traditional total hip arthroplasty, including more accurate restoration of native hip biomechanics, lower incidence of limb length discrepancy, lower dislocation rates, preservation of proximal femoral bone stock and high rate of return to high demand activities, including high impact running and cutting sports.
Leg length discrepancy and changes in offset are associated with pain, limp, patient dissatisfaction and litigation after hip arthroplasty (14). Several studies comparing total hip arthroplasty and surface replacement arthroplasty have demonstrated more accurate restoration of native hip biomechanics with hip resurfacing. Three separate single institution retrospective studies comparing surface replacement arthroplasty (SRA) to total hip arthroplasty (THA) patients all found that radiographic offset and leg length were more accurately restored with hip resurfacing arthroplasty than total hip arthroplasty (15-17). These results indicate an advantage of SRA over THA in restoration of native biomechanics that may impact patient function and satisfaction.
The incidence of dislocation after primary total hip arthroplasty ranges from 0.3% to 10% and may lead to the need for revision surgery (18). The metal-on-metal bearing used in modern hip resurfacing allows for the use of larger femoral heads than total hip arthroplasty. As a result, dislocations are rare after SRA with an incidence of around 0.5%, lower than after primary THA (19,20).
Several studies have investigated the bone density of the proximal femur after THA and SRA. The extent of proximal femoral stress shielding after primary THA is dependent on the design of the implant, but is universally present after primary THA. SRA, however, provides more physiologic loading of the proximal femur with decreased stress shielding, leading to an increase in bone mineral density of the proximal femur postoperatively (19,21,22). The stress shielding is of clinical significance apparently since pain drawing studies indicate thigh pain in total hip arthroplasty is about three times greater than in SRA (23).
SRA also has a potential functional advantage over THA. A recent systematic review by Hellman et al. found that compared to THA patients, SRA patients demonstrated superior UCLA activity scores, increased return to heavy activity including sport and manual labor, less thigh pain and superior quality of life scores. Although Harris Hip Scores were not significantly different between SRA and THA in the studies included, the authors concluded that SRA has the potential to provide improved outcomes in active individuals (24).
Success depends on implant design
Several modern hip resurfacing designs have been utilized over the past 20 years (Table 1). The vast majority have been recalled or removed from the market due to high failure rates. Medical device approval processes failed to detect manufacturing and design specific features that made some implants more prone to catastrophic wear and failure. As a result, several SRA designs were approved for clinical use and were in widespread use before these issues were recognized.
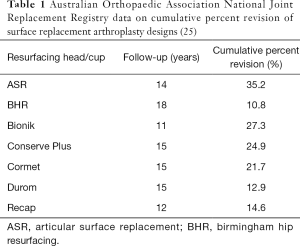
Full table
At the time of development of most modern hip resurfacing designs, medical device approval processes in Europe and the United States allowed for the approval of use of devices with proven equivalence to similar devices already in clinical use. Unfortunately, the in vitro wear simulator testing used as a major part of this pre-market approval process failed to accurately mimic in vitro conditions. Specifically, the simulators relied on high frequency, continuous conditions, leading to exaggerated lubrication conditions that artificially protected the bearings from wear (25). These tests were not reflective of daily activity patterns that can break down the fluid-film lubrication in vivo and put the wear characteristics of the actual bearing surface material (25). As a consequence, the low bearing wear rates seen in the simulators did not reflect the actual in vivo high wear rates for certain implants that led to high clinical failure rates. The components were also more sensitive to malposition than anticipated.
Manufacturing processes varied amongst different implant designs. The successful metal-on-metal total hip arthroplasties that the metal on metal SRAs were based on were high carbon CoCr alloy manufactured as-cast without any post-casting heat treatment. In attempts to improve mechanical properties and streamline manufacturing, several implant companies introduced post-casting thermal treatments that had the unrecognized effect of leaching carbides out of the alloy, which led to unfavorable wear characteristics. As-cast, high carbon SRA designs i.e., BHR and Conserve Plus have continued to perform well with good survivorship.
Implant specific design features also led to diversity in failure rates amongst the different SRA models. Cup coverage angles were reduced in several designs in an effort to increase impingement free range of motion. However, this had the unintended consequence of increasing propensity for edge loading and wear (26). The DePuy ASR, for example, had a shallower cup than other designs with a coverage angle of compared to the Conserve Plus and the BHR. The higher failure rates reported for the smaller size BHR components was related in part to the lower associated coverage angles and these components were subsequently removed from the market.
Although bone conserving on the femoral side, SRA requires reaming to large cup sizes to accommodate the appropriate femoral shell size. To improve conservation of acetabular bone, some manufacturers made their cups thinner. In hard bone, these thin cups deflected and deformed during insertion, leading to the conversion of polar bearings to equatorial bearings resulting in loss of fluid-film lubrication, seizing and runaway wear. For example, the 50 mm diameter DePuy Pinnacle THA acetabular shell has a thickness of 5.25 mm at the rim, where the ASR only had a thickness of 3.5 mm at the same location.
Diametral clearance between the head and cup similarly varied between designs. Perfect 1:1 congruity between the components leads to equatorial seizing bearing, leading to high friction and wear. Similarly, high clearance designs where there is imperfect congruity between the components can lead to higher wear from imperfect motion and sliding of the ball in the cup. Therefore, the ideal design has a low clearance but not perfect congruity. This allows for a large polar contact area with a small peripheral inlet area where fluid can enter the bearing and provide fluid film lubrication (27). Implants designed with extremely low clearances in attempt to improve wear properties failed at higher rates due to deformation of the cup on insertion leading to total contact or equatorial bearings or manufacturing variabilities that led to some devices with clearances too small to allow adequate fluid film lubrication to occur.
Finally, design features of the individual acetabular or femoral components led to high failure rates in those designs. For instance, the Zimmer Durom acetabular shell failed at an unacceptably high rate due to the peripheral press fit and sharp peripheral fins that prevented contact between the porous plasma sprayed surface of the shell and the prepared host bone (28).
Patient selection: narrow indications for successful outcomes
Modern criteria for SRA have been developed based on high failure rates observed in certain populations. The best survivorship is seen in males less than 60 years of age with osteoarthritis and a femoral head component size greater than or equal to 48 mm (29). Most surgeons avoid performing the procedure in women of childbearing age as there remains concerns for systemic absorption of metal ions that can cross the placenta in women who may become pregnant. Women also have a higher revision risk for several reasons (30). First, their hips are on average smaller than males. Smaller component sizes leave a smaller margin for error in component positioning, with each degree of acetabular component malposition leading to a larger increase in edge loading than larger component sizes. Also, the dysplasia and increased femoral and acetabular anteversion increases the risk of component positioning that is prone to edge loading. Additionally, bone quality on average is weaker in women than men, leading to a higher risk of femoral neck fractures postoperatively. Similarly, older age is a risk factor for femoral neck fractures due to osteopenic or osteoporotic bone. Patients with avascular necrosis should be approached with caution as they have a higher risk of femoral neck fracture and femoral component failure from aseptic loosening unless very strict selection criteria are applied (31).
Surgeon experience matters
SRA is a technically demanding procedure with is a significant early learning curve of cases associated with a higher incidence of early complications (32,33). Although the anatomy may be familiar, the exposure can be quite challenging for inexperience surgeons. Achieving adequate visualization and access to the acetabulum with the intact femoral head and neck can be difficult. If acetabular exposure is inadequate, bony preparation, full component seating and component position will likely be compromised. Aseptic loosening or edge loading may result. Similarly, delivery of the femoral head out of the wound for preparation of the femoral component can be difficult for a surgeon inexperienced with the releases required for adequate exposure. This may lead to improper sizing of the component, femoral neck notching, perforation, excessive anteversion or retroversion, varus alignment, all of which can increase the risk for femoral neck fracture.
The learning curve for accurate component position may be as long as 50 cases (26,34). Acetabular component position is the single most important factor leading to successful long term outcomes with SRA (35). Excessive anteversion and inclination lead to edge loading and increased wear and must be avoided. Additionally, the cup must be medialized and anteverted enough to avoid anterior overhang of the rim of the cup that may lead to iliopsoas impingement on the sharp edge of the cup.
Conclusions
SRA remains an excellent option for hip reconstruction in patients who wish to return to high levels of activity with demonstrated benefits over THA in this select group. History has taught us that the details of implant design features and manufacturing processes, patient selection and surgeon experience are paramount for achieving successful long-term results. As SRA enters its next generation of implants with non-metal-on-metal bearings, these lessons should be applied to critically evaluate the risks, benefits, techniques and indications for the new designs.
Acknowledgments
Funding: None.
Footnote
Provenance and Peer Review: This article was commissioned by the Guest Editors (George Grammatopoulos and Paul E. Beaulé) for the series “Hip Resurfacing for the Young Arthritic Hip” published in Annals of Joint. The article has undergone external peer review.
Conflict of Interest: Both authors have completed the ICMJE uniform disclosure form (available at http://dx.doi.org/10.21037/aoj.2019.12.08). The series “Hip Resurfacing for the Young Arthritic Hip” was commissioned by the editorial office without any funding or sponsorship. CML reports consulting fees from Zimmer-Biomet, MicroPort Orthopedics and Medtronic outside the submitted work. BRL Barrack reports royalties and consulting fees from Stryker, grants from Zimmer-Biomet, grants from EOS Imaging, grants from Smith & Nephew, grants from Wright Medical Technology, other from The McGraw-Hill Companies, Inc, other from Wolters Kluwer Health - Lippincott Williams & Wilkins, outside the submitted work. The authors have no other conflicts of interest to declare.
Ethical Statement: The authors are accountable for all aspects of the work in ensuring that questions related to the accuracy or integrity of any part of the work are appropriately investigated and resolved.
Open Access Statement: This is an Open Access article distributed in accordance with the Creative Commons Attribution-NonCommercial-NoDerivs 4.0 International License (CC BY-NC-ND 4.0), which permits the non-commercial replication and distribution of the article with the strict proviso that no changes or edits are made and the original work is properly cited (including links to both the formal publication through the relevant DOI and the license). See: https://creativecommons.org/licenses/by-nc-nd/4.0/.
References
- Smith-Petersen MN. Evolution of mould arthroplasty of the hip joint. J Bone Joint Surg Br 1948;30B:59-75. [Crossref] [PubMed]
- Charnley J. Total hip replacement by low-friction arthroplasty. Clin Orthop Relat Res 1970;7-21. [PubMed]
- Sharp DJ, Porter KM. The Charnley total hip arthroplasty in patients under age 40. Clin Orthop Relat Res 1985;51-6. [PubMed]
- Amstutz HC, Clarke IC, Christie J, et al. Total hip articular replacement by internal eccentric shells: the "tharies" approach to total surface replacement arthroplasty. Clin Orthop Relat Res 1977;261-84. [PubMed]
- Capello WN, Misamore GW, Trancik TM. The Indiana conservative (surface-replacement) hip arthroplasty. J Bone Joint Surg Am 1984;66:518-28. [Crossref] [PubMed]
- Capello WN, Misamore GW, Trancik TM. Conservative total hip arthroplasty. Orthop Clin North Am 1982;13:833-42. [PubMed]
- Amstutz HC, Dorey F, O'Carroll PF. THARIES resurfacing arthroplasty. Evolution and long-term results. Clin Orthop Relat Res 1986;92-114. [PubMed]
- Kim WC, Grogan T, Amstutz HC, et al. Survivorship comparison of THARIES and conventional hip arthroplasty in patients younger than 40 years old. Clin Orthop Relat Res 1987;269-77. [PubMed]
- Amstutz HC, Kabo M, Hermens K, et al. Porous surface replacement of the hip with chamfer cylinder design. Clin Orthop Relat Res 1987;140-60. [PubMed]
- Schmalzried TP GD, Grecula M, Amstutz HC. The relationship between the design, position, and articular wear of acetabular components in-serted without cement and the development of pelvic osteolysis. J Bone Joint Surg Am 1994;76:677-88. [Crossref] [PubMed]
- Weber BG. Experience with the Metasul total hip bearing system. Clin Orthop Relat Res 1996;S69-77. [Crossref] [PubMed]
- Wagner M, Wagner H. Preliminary results of uncemented metal on metal stemmed and resurfacing hip replacement arthroplasty. Clin Orthop Relat Res 1996;S78-88. [Crossref] [PubMed]
- McMinn D, Treacy R, Lin K, et al. Metal on metal surface replacement of the hip. Experience of the McMinn prothesis. Clin Orthop Relat Res 1996;S89-98. [Crossref] [PubMed]
- Pyrko P, Zuckerman J. Leg Length Discrepancy in Primary Total Hip Arthroplasty. Bull Hosp Jt Dis 2013;2016:82-7. [PubMed]
- Parry MC, Povey J, Blom AW, et al. Comparison of Acetabular Bone Resection, Offset, Leg Length and Post Operative Function Between Hip Resurfacing Arthroplasty and Total Hip Arthroplasty. J Arthroplasty 2015;30:1799-803. [Crossref] [PubMed]
- Brown NM, Foran JR, Della Valle CJ. Hip resurfacing and conventional THA: comparison of acetabular bone stock removal, leg length, and offset. Orthopedics 2013;36:e637-641. [Crossref] [PubMed]
- Ahmad R, Gillespie G, Annamalai S, et al. Leg length and offset following hip resurfacing and hip replacement. Hip Int 2009;19:136-40. [Crossref] [PubMed]
- Werner BC, Brown TE. Instability after total hip arthroplasty. World J Orthop 2012;3:122-30. [Crossref] [PubMed]
- Gerhardt DM, Smolders JM, Rijnders TA, et al. Changes in bone mineral density and femoral neck narrowing in the proximal femur three to five years after hip resurfacing versus conventional total hip arthroplasty. J Arthroplasty 2015;30:308-14. [Crossref] [PubMed]
- Arndt JM, Wera GD, Goldberg VM. An initial experience with hip resurfacing versus cementless total hip arthroplasty. HSS J 2013;9:145-9. [Crossref] [PubMed]
- Huang Q, Shen B, Yang J, et al. Changes in bone mineral density of the acetabulum and proximal femur after total hip resurfacing arthroplasty. J Arthroplasty 2013;28:1811-5. [Crossref] [PubMed]
- Penny JO, Brixen K, Varmarken JE, et al. Changes in bone mineral density of the acetabulum, femoral neck and femoral shaft, after hip resurfacing and total hip replacement: two-year results from a randomised study. J Bone Joint Surg Br 2012;94:1036-44. [Crossref] [PubMed]
- Nam D, Nunley RM, Sauber TJ, et al. Incidence and Location of Pain in Young, Active Patients Following Hip Arthroplasty. J Arthroplasty 2015;30:1971-5. [Crossref] [PubMed]
- Hellman MD, Ford MC, Barrack RL. Is there evidence to support an indication for surface replacement arthroplasty?: a systematic review. Bone Joint J 2019;101-B:32-40. [Crossref] [PubMed]
- Australian Orthopaedic Association National Joint Replacement Registry Annual Report. Adelaide: AOA, 2019.
- Kamali A, Hussain A, Li C, et al. Tribological performance of various CoCr microstructures in metal-on-metal bearings: the development of a more physiological protocol in vitro. J Bone Joint Surg Br 2010;92:717-25. [Crossref] [PubMed]
- De Haan R, Pattyn C, Gill HS, et al. Correlation between inclination of the acetabular component and metal ion levels in metal-on-metal hip resurfacing replacement. J Bone Joint Surg Br 2008;90:1291-7. [Crossref] [PubMed]
- Beaulé PE, Mussett SA, Medley JB. Metal-on-Metal Bearings in Total Hip Arthroplasty. Instr Course Lect 2010;59:17-25. [PubMed]
- Long WT, Dastane M, Harris MJ, et al. Failure of the Durom Metasul acetabular component. Clin Orthop Relat Res 2010;468:400-5. [Crossref] [PubMed]
- Ford MC, Hellman MD, Kazarian GS, et al. Five to Ten-Year Results of the Birmingham Hip Resurfacing Implant in the U.S.: A Single Institution's Experience. J Bone Joint Surg Am 2018;100:1879-87. [Crossref] [PubMed]
- . Canadian Arthroplasty S. The Canadian Arthroplasty Society's experience with hip resurfacing arthroplasty. An analysis of 2773 hips. Bone Joint J 2013;95-B:1045-51. [Crossref] [PubMed]
- Pyda M, Koczy B, Widuchowski W, et al. Hip resurfacing arthroplasty in treatment of avascular necrosis of the femoral head. Med Sci Monit 2015;21:304-9. [Crossref] [PubMed]
- Berend KR, Lombardi AV Jr, Adams JB, et al. Unsatisfactory surgical learning curve with hip resurfacing. J Bone Joint Surg Am 2011;93:89-92. [Crossref] [PubMed]
- Nunley RM, Zhu J, Brooks PJ, et al. The learning curve for adopting hip resurfacing among hip specialists. Clin Orthop Relat Res 2010;468:382-91. [Crossref] [PubMed]
- O'Neill M, Beaule PE, Bin Nasser A, et al. Canadian academic experience with metal-on-metal hip resurfacing. Bull NYU Hosp Jt Dis 2009;67:128-31. [PubMed]
Cite this article as: Lawrie CM, Barrack RL. Hip resurfacing arthroplasty—what has history taught us? Ann Joint 2020;5:20.