The biomechanics of current reverse shoulder replacement options
The biomechanics of current reverse shoulder replacement options
Since the introduction of reverse shoulder arthroplasty (RSA) over 40 years ago, many prosthesis designs have endeavored to improve shoulder pain and function in patients with challenging conditions such as massive rotator cuff tears, cuff tear arthropathy, proximal humerus fractures, and inflammatory arthropathy. While sharing the common design of an inverted or “reverse” ball-and-socket joint, these designs have exhibited many variations in geometry. Even today RSA designs remain divergent in many aspects. Presently the shoulder arthroplasty surgeon has myriad choices for prosthesis geometry, including size, shape, position of the glenoid component, humeral neck angle, on-lay vs. in-lay, constrained liners, etc. There are over 30 RSA systems available, with multiple configurations within each system (1). Furthermore, the anatomic and physiologic substrate of each patients’ shoulder must be considered. Some may have intact or salvageable cuff tendons, the deltoid may be compromised, and osteopenic or osteoporotic bone may be present. With so many prosthesis choices and patient variables, it can be daunting to objectively determine the optimal prosthesis geometry and implantation technique for a given patient. Some surgeons may have the attitude that RSA is more “forgiving” or tolerant of sub-optimal implant selection or technique. In this scenario, the surgeon may be missing an opportunity to perform the best operation for the patient and rather settling for a good operation. In recent years there have been many biomechanical studies of RSA that provide guidance. As RSA has grown from the realm of salvage operation to mainstream orthopedics, savvy patients are more demanding of good outcomes, and surgeons should be more discerning. The aim of this review article is to present an update of recent RSA biomechanical studies that are pertinent to prosthesis design and surgical technique with an emphasis on how these factors affect shoulder function and prosthesis performance. All results must be interpreted in the context of the prosthesis geometry that is being studied. Papers were included in this review utilizing cadaver simulation, computer simulation, radiographic review, and clinical papers with biomechanical conclusions.
Prosthesis geometry—classification systems
In their paper proposing a RSA classification system, Routman et al. define glenoid prosthesis designs having the center of rotation (COR) 5 mm or less from the glenoid face as medialized glenoid (MG) designs and those with COR greater than 5 mm from the face as lateralized glenoid (LG) designs (2) (Figure 1). MG designs shift the COR to a position that is more medial than the native anatomy. This improves fixation by decreasing shear stress and it imparts a greater moment arm to the deltoid which reduces the deltoid force required for abduction. MG designs potentially shorten and weaken residual rotator cuff muscles and produce less deltoid wrapping which contributes to instability. LG designs medialize the COR to a lesser degree than MG designs, resulting in comparatively shorter deltoid moment arms and a less efficient deltoid. LG designs have the potential to better restore rotator cuff tension compared to MG designs, and LG designs increase deltoid wrapping to a greater degree than MG designs. The authors propose that RSA designs with humeral component offset of 15 mm or less be designated medialized humerus (MH) designs, and designs with humeral component offset of greater than 15 mm be designated lateralized humerus designs. MH designs shorten rotator cuff muscle length and decrease deltoid wrapping, whereas LH designs improve rotator cuff tension and increase deltoid wrapping. Utilizing a computer model, they report on the deltoid wrapping angle for the normal intact shoulder (48°), a MG/MH design (8°), LG/LH designs (28°), and several MG/LH designs (24°–80°).
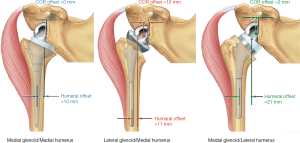
Werthel (1) proposes a modification to the RSA classification nomenclature described by Hamilton et al. (3) and Routman et al. (2). He determines glenoid lateralization, humeral lateralization, and global lateralization for 22 prostheses and describes humeral lateralization as medialized, minimally lateralized, lateralized, or highly lateralized. As an illustration of the range of RSA configurations available, one of the systems he analyzed is capable of achieving global lateral offset of 3.3 to 20.9 mm. In this classification, global offset is influenced by prosthesis geometry as well as surgical technique (neck-shaft angle and in-lay vs. on-lay). Although the contribution of neck-shaft angle to lateralization of the humerus is minimal at +3.2 mm for 135° compared to 155°. Whether the design is inlay or on-lay seems to have a greater effect on humeral lateralization, and all the lateralized humerus and highly lateralized humerus prostheses are on-lay designs (1).
Boutsiadis et al. describe lateralization and distalization shoulder angles, easily measured from anteroposterior shoulder radiographs. These angles account for shoulder anatomy, prosthesis geometry and surgical technique. Active external rotation was improved for lateralization shoulder angles between 75° and 95°. Distalization shoulder angles between 40° and 65° correlated with increased active forward flexion (4).
Prosthesis geometry—position of the COR
The glenoid articulating component of RSA, or glenosphere, is typically a spherical or ovoid section with radius of curvature from 32 to 46 mm. One of the design elements of Grammont’s Delta III RSA that lead to improved glenoid fixation was medialization of the COR. This was accomplished by designing the glenosphere as ½ of a sphere, resulting in the geometric center of the implant being coincident with the surface of the native glenoid bone (5). Earlier iterations of RSA designs had more lateral COR’s which produced greater shear torque at the glenoid prosthesis-bone interface and subsequent high failure rates due to loosening of the glenoid component.
RSA designs inherently alter the deltoid and rotator cuff moment arms from anatomic based on the geometry of the prosthesis. In a sophisticated integrated kinematic and finite-element-analysis computer model, Liou and colleagues compared the effects of prosthesis design—specifically humeral lateralized design, glenoid lateralized design, and Grammont design—on deltoid and rotator cuff muscle force requirements for shoulder abduction and forward flexion (6). Their main conclusion was that the position of the COR relative to the glenoid face and the amount of humeral lateralization independently influence deltoid efficiency.
Medial glenoid RSA designs also increase the deltoid moment (or lever) arm to a greater extent than do lateral glenoid designs, reducing the deltoid force required for shoulder elevation (3,5). For medial glenoid/medial humeral designs, lowering of the COR and lengthening of the deltoid seemed to be important for retensioning the deltoid (5). Ferrier et al. suggest that for a Grammont-style prosthesis humeral lowering of at least 24 mm is necessary (7). Although a clinical follow-up study could not correlate deltoid lengthening with improved forward elevation (8).
In contrast, a retrospective analysis of 68 RSA’s, Müller et al. found 12° greater external rotation in adduction and greater abduction strength in patients with 44 mm glenospheres compared to 36 mm glenospheres (9). This study utilized a 155° inlay medial humeral offset prosthesis design which may explain the disparate results for ROM.
In a computer model examining the effect of glenosphere configurations, Lädermann et al. show that an eccentric (2 mm inferior offset) 36 mm glenosphere provides better impingement-free ROM compared to neutral 36 mm, neutral 42 mm, 10° tilted 36 mm, and 10 mm BIO-RSA 36 mm glenospheres (10). These results are for a 145° on-lay prosthesis design.
Prosthesis geometry—glenosphere size
RSA stability may be improved with use of a larger diameter glenosphere; however, this may come at the expense of increased polyethylene wear. Haggart et al. demonstrated results that mirror results in total hip arthroplasty—larger glenospheres caused greater polyethylene volume loss and volumetric wear rates. Smaller glenospheres had greater polyethylene surface deviations (11).
In one study, a 4-mm eccentric glenosphere reduced the rate of grade 2 scapular notching for a medial glenoid/medial humeral inlay design (12). One clinical study showed that 42-mm glenospheres were related to significantly higher outcome scores and better postoperative active external rotation for a medial glenoid/lateral humeral design (13).
A retrospective analysis of 68 RSA’s, Müller et al. found 12° greater external rotation in adduction and greater abduction strength in patients with 44-mm glenospheres compared to 36-mm glenospheres (9). This study utilized a 155° inlay medial humeral offset prosthesis design which may explain the disparate results for ROM.
Glenoid version and humeral polyethylene insert rotation were modelled using finite element analysis (FEA) to investigate impingement of the insert on the inferior glenoid/scapula and subsequent subluxation. It was found that for the on-lay lateral humeral design studied, neutral glenoid version caused the least degree of subluxation. Posterior rotation of the humeral insert also reduced subluxation while anterior rotation of the humeral insert increased subluxation. The authors caution that variability in prosthesis design and the local soft tissue environment must also be considered when deciding on humeral implant rotation for a given patient (14).
Prosthesis geometry—humeral component
Many RSA systems available today are modular. The surgeon can choose prosthesis components that result in various amounts of humeral offset. Walker et al. showed that the deltoid moment arms are very strongly affected by the joint center and humeral offset with changes of at least 5, 12, and 6 mm for the anterior, lateral, and posterior deltoid moment arms respectively (15). Based on the results of their computer model, they recommend subject-specific treatment planning for RSA. They noted that deltoid moment arm variations were not consistent across all subjects, suggesting that shoulder size, native shoulder anatomy, and individual kinematics also affect moment arms.
A computer simulation of a medial glenoid/lateral humerus on-lay design showed the effect of changing the neck angle from 145° to 135° was improvement in adduction (+6.5°), extension (+42.3°), and internal rotation (7°) for the 2 mm eccentric glenoid configuration (16). Abduction and forward flexion were not affected.
For a medial glenoid/medial humeral design, one clinical study did not show any difference in ROM for neutral humeral version implantation technique vs. 20° retroversion technique (17).
There have been few studies investigating optimal humeral version. Kontaxis et al. simulated activities of daily living (ADLs) in 30 shoulders using computer simulation (18). They found that version affected the location of extra-articular and intra-articular impingement during ADLs and range of motion (ROM) in standard activities. Retroversion was associated with greater ROM, but it also caused impingement between the greater tuberosity and the coracoid during ADLs and it increased the risk of contact between the anterior glenoid and the humeral cup. Humeral anteversion was associated with increased contact of the humeral cup and the inferior scapular border and impingement between the greater tuberosity and the acromion. The authors suggest that neutral humeral version should be preferred. It is noted that RSA prosthesis geometry was constant for this study and the design was a lateral humeral on-lay prosthesis with a medial glenoid. Thus, the results of this study may not extrapolate to other prosthesis geometries.
The FEA study by Langohr et al. investigated the effect of neck-shaft angle, glenosphere size, and cup depth on articular contact stress (19). Lower neck shaft angle, larger glenosphere diameter, and decreased cup depth all improve RSA ROM but also alter joint kinetics—with predominantly negative effects. Decreasing the neck shaft angle from 155° to 135° decreased the average joint contact area by 59% and increased the maximum contact stress by 286%. Increasing the glenosphere diameter from 38 to 42 mm increased the contact area by 12% but only decreased the maximum contact stress by 2%. Increasing the cup depth from normal to deep resulted in 52% increased surface area and 36% reduction in maximum stress. The authors acknowledge the limitations of their study such as not accounting for the increase humeral offset which is seen in many 135° neck shaft angle RSA designs. Increased deltoid wrapping may offset the increased stress seen in these RSA designs.
To answer the question of whether RSA designs with higher constraint polyethylene components come at the expense of ROM, Abdulla et al. performed a cadaver experiment using a shoulder simulator and three different degrees of constraint in a medial glenoid/custom humeral design with 155° neck angle (20). They demonstrated no loss of abduction ROM amongst the different liner constraint designs and stated impingement occurred rather between the acromion and tuberosity as the COR was constant between the constraint conditions. However, active external rotation was decreased for the constrained designs vs. the non-constrained design. Furthermore, the resultant joint load was not significantly altered by increasing the constraint of the polyethylene component.
Humeral lateralization was identified as an important parameter to improve joint and muscle loading in a cadaver model (21). This study showed that increasing humeral laterization from 0 to 10 mm decreased the deltoid muscle force required for abduction from 68% body weight (BW) to 65% BW. Glenosphere lateralization from 0 to 10 mm increased the deltoid force required for abduction from 61% BW to 70% BW. Similarly, increasing polyethylene cup thickness also increased the required deltoid force.
In a retrospective study of 75 patients who underwent RSA using a medial glenoid/medial humeral offset design, poor active anterior shoulder elevation was found in a subset of patients (22). The authors identified excessive lateral offset in these patients resulting from high humeral head cuts, insufficient humeral stem insertion, and thicker liner insertion. They caution against intraoperative humeral lateralization of medial humeral prostheses.
Berton et al. used a computer model to evaluate the effect of humeral implant version on teres major moment arm and muscle length (23). They report that 40° humeral retroversion corresponded to the greatest teres minor muscle length but the smallest moment arm. Anteverted fixation of the prosthesis to the humerus resulted in the greatest moment arm for the teres minor yet the shortest muscle lengths. They suggest 0°–20° as an appropriate compromise. It is important to note that the prosthesis geometry for this study was MG/MH and extrapolation of these results to other designs may not be appropriate.
Bone integrity considerations
To determine how much glenoid bone stock is necessary for initial fixation of the glenoid component of RSA, Martin et al. tested multiple degrees of superior glenoid bone deficiency in a bone foam model. Bone support of 50% showed significantly greater superior, medial, and total baseplate micromotion compared to the 75%, 90%, and full support conditions (24).
Stress at the baseplate and displacement of the baseplate were predicted by a 3D FEA to be lower with smaller glenospheres (16). This same study also showed lower stress and less displacement for lateralization of the glenoid by the prosthesis compared to lateralization with bone graft. They suggest that bony lateralization be limited to 5 mm.
Glenoid version, glenoid bone loss, bone grafting of the glenoid, and prosthesis design determine lateralization of the COR. Concentric or eccentric glenoid bone loss may be present, typically located posteriorly or postero-superiorly. Many contemporary RSA systems offer flexible options for glenoid augmentation and/or use of a lateralizing glenosphere. An FEA model demonstrated that bone lateralization from the glenoid face utilizing greater than 5 mm of bone graft raised displacement above the 150 µm threshold thought to be the upper limit tolerable for osseous integration. Prosthetic lateralization of 10 mm was found to have acceptable displacement (25).
To investigate the effect of glenosphere implant position on acromial stress and possibly subsequent acromial stress fractures, Wong et al. developed a sophisticated computer model utilizing reconstructed cadaveric shoulders with 38 mm glenospheres and 155° neck angle humerus component, kinematic modeling, and FEA of acromial stress distribution for active abduction from 0°–120° (26). This model incorporated the deltoid wrapping effect of contact between the proximal humerus and the deep surface of the deltoid by which the deltoid compresses the joint and the force vector of the deltoid is displaced by the tuberosity at low abduction angles. They concluded that glenosphere positioning has an important role in the stress generated in the acromion after RSA with 5 mm glenosphere inferiorization decreasing maximum principal acromial stress by 2.6%. Glenosphere lateralization of 10 mm increased maximum principal acromial stress by 17.2%. Humeral lateralization of 5 mm did not cause significant acromial stress increase. The authors suggest that the optimal configuration to minimize acromial stress for this implant geometry is inferiorization without lateralization of the glenosphere with medialization of the humerus.
Similar results were found in a cadaver model utilizing an eccentric glenosphere that is equivalent biomechanically to moving the glenosphere inferiorly (27). Compared to the non-eccentric glenosphere, less deltoid force was required for abduction which may relate to lower acromial stress.
Rotator cuff considerations
At mean follow-up of 30 months s/p RSA, 10 of 25 repaired subscapularis tendons were intact on ultrasound evaluation. There was no measurable difference between the subscapularis repair group and the subscapularis deficient group for dislocation, ROM, or outcomes scores (28).
This effect of deltoid wrapping was further investigated in a cadaver study wherein the authors conclude that humeral lateralization produced a stabilizing compressive joint load without increasing joint load magnitude, or in other words deltoid wrapping changes the direction of the joint force vector to make it more perpendicular to the glenoid without increasing the magnitude of the joint force vector (29). In this same study the authors find that concomitant rotator cuff repair increases the deltoid force required for abduction, especially for lateral humerus design RSA prostheses which saw 29% increase in joint load. Thus, they suggest that humeral lateralization may be used in place of rotator cuff repair.
Although RSA is indicated for massive cuff tears, often the surgeon will find some of the rotator cuff tendons intact and functional. RSA function seems to be better when the posterior cuff is intact. Prosthesis geometry and surgical technique can greatly distort the anatomic rotator cuff line-of action by displacing the cuff insertions on the lesser and greater tuberosities. Chan et al. suggest from a cadaver simulator experiment that humeral lateralization improves rotator cuff torque for some arm positions and may be beneficial for improving strength after RSA (30). Their experiment utilized a 155° neck design.
Conclusions
RSA technology has undergone a rapid window of design development since the modern rebirth of this prosthesis starting with Grammont in an effort to maximize ROM and minimize design-related complications. Additionally, the hyper-modularity of RSA devices currently being offered to surgeons requires a working knowledge of the biomechanical consequences of design decisions for those considering performing RSA. There is little long-term follow demonstrating meaningful clinical outcomes comparing design options, so surgeons should move cautiously with these biomechanical concepts in mind.
Acknowledgments
Funding: None.
Footnote
Provenance and Peer Review: This article was commissioned by the Guest Editor (Joseph A. Abboud) for the series “Evolving Trends in Reverse Shoulder Arthroplasty” published in Annals of Joint. The article has undergone external peer review.
Conflict of Interest: The series “Evolving Trends in Reverse Shoulder Arthroplasty” was commissioned by the editorial office without any funding or sponsorship. MLH and HR are consultants for Exactech. The authors have no other conflicts of interest to declare.
Ethical Statement: The authors are accountable for all aspects of the work in ensuring that questions related to the accuracy or integrity of any part of the work are appropriately investigated and resolved.
Open Access Statement: This is an Open Access article distributed in accordance with the Creative Commons Attribution-NonCommercial-NoDerivs 4.0 International License (CC BY-NC-ND 4.0), which permits the non-commercial replication and distribution of the article with the strict proviso that no changes or edits are made and the original work is properly cited (including links to both the formal publication through the relevant DOI and the license). See: https://creativecommons.org/licenses/by-nc-nd/4.0/.
References
- Werthel JD. Humeral lateralization: What is it, is it really useful? In: Boileau P. editor. Shoulder Concepts 2018: Arthroplasty for the Young Arthritic Shoulder. Montpellier, France: Sauramps Médical, 2018:251-61.
- Routman HD, Flurin PH, Wright TW, et al. Reverse Shoulder Arthroplasty Prosthesis Design Classification System. Bull Hosp Jt Dis 2013;2015:S5-14. [PubMed]
- Hamilton MA, Diep P, Roche C, et al. Effect of reverse shoulder design philosophy on muscle moment arms. J Orthop Res 2015;33:605-13. [Crossref] [PubMed]
- Boutsiadis A, Lenoir H, Denard PJ, et al. The lateralization and distalization shoulder angles are important determinants of clinical outcomes in reverse shoulder arthroplasty. J Shoulder Elbow Surg 2018;27:1226-34. [Crossref] [PubMed]
- Boileau P, Watkinson DJ, Hatzidakis AM, et al. Grammont reverse prosthesis: design, rationale, and biomechanics. J Shoulder Elbow Surg 2005;14:147S-161S. [Crossref] [PubMed]
- Liou W, Yang Y, Petersen-Fitts GR, et al. Effect of lateralized design on muscle and joint reaction forces for reverse shoulder arthroplasty. J Shoulder Elbow Surg 2017;26:564-72. [Crossref] [PubMed]
- Ferrier A, Blasco L, Marcoin A, et al. Geometric modification of the humeral position after total reverse shoulder arthroplasty: What is the optimal lowering of the humerus? J Shoulder Elbow Surg 2018;27:2207-13. [Crossref] [PubMed]
- Sabesan VJ, Lombardo D, Josserand D, et al. The effect of deltoid lengthening on functional outcome for reverse shoulder arthroplasty. Musculoskelet Surg 2016;100:127-32. [Crossref] [PubMed]
- Müller AM, Born M, Jung C, et al. Glenosphere size in reverse shoulder arthroplasty: Is larger better for external rotation and abduction strength? J Shoulder Elbow Surg 2018;27:44-52. [Crossref] [PubMed]
- Lädermann A, Denard PJ, Boileau P, et al. What is the best glenoid configuration in onlay reverse shoulder arthroplasty? Int Orthop 2018;42:1339-46. [Crossref] [PubMed]
- Haggart J, Newton MD, Harner S, et al. Neer Award 2017: Wear rates of 32-mm and 40-mm glenospheres in a reverse total shoulder arthroplasty wear simulation model. J Shoulder Elbow Surg 2017;26:2029-37. [Crossref] [PubMed]
- Choi CH, Kim SG, Lee JJ, et al. Comparison of clinical and radiological results according to glenosphere position in reverse total shoulder arthroplasty: A short-term follow-up study. Clin Orthop Surg 2017;9:83. [Crossref] [PubMed]
- Mollon B, Mahure SA, Roche CP, et al. Impact of glenosphere size on clinical outcomes after reverse total shoulder arthroplasty: An analysis of 297 shoulders. J Shoulder Elbow Surg 2016;25:763-71. [Crossref] [PubMed]
- Permeswaran VN, Caceres A, Goetz JE, et al. The effect of glenoid component version and humeral polyethylene liner rotation on subluxation and impingement in reverse shoulder arthroplasty. J Shoulder Elbow Surg 2017;26:1718-25. [Crossref] [PubMed]
- Walker DR, Kinney AL, Wright TW, et al. How sensitive is the deltoid moment arm to humeral offset changes with reverse total shoulder arthroplasty? J Shoulder Elbow Surg 2016;25:998-1004. [Crossref] [PubMed]
- Werner BS, Chaoui J, Walch G. The influence of humeral neck shaft angle and glenoid lateralization on range of motion in reverse shoulder arthroplasty. J Shoulder Elbow Surg 2017;26:1726-31. [Crossref] [PubMed]
- de Boer FA, van Kampen PM, Huijsmans PE. Is there any influence of humeral component retroversion on range of motion and clinical outcome in reverse shoulder arthroplasty? A clinical study. Musculoskelet Surg 2017;101:85-9. [Crossref] [PubMed]
- Kontaxis A, Chen X, Berhouet J, et al. Humeral version in reverse shoulder arthroplasty affects impingement in activities of daily living. J Shoulder Elbow Surg 2017;26:1073-82. [Crossref] [PubMed]
- Langohr GD, Willing R, Medley JB, et al. Contact mechanics of reverse total shoulder arthroplasty during abduction: the effect of neck-shaft angle, humeral cup depth, and glenosphere diameter. J Shoulder Elbow Surg 2016;25:589-97. [Crossref] [PubMed]
- Abdulla I, Langohr DG, Giles JW, et al. The effect of humeral polyethylene insert constraint on reverse shoulder arthroplasty biomechanics. Shoulder Elbow 2018;10:25-31. [Crossref] [PubMed]
- Giles JW, Langohr GD, Johnson JA, et al. Implant Design Variations in Reverse Total Shoulder Arthroplasty Influence the Required Deltoid Force and Resultant Joint Load. Clin Orthop Relat Res 2015;473:3615-26. [Crossref] [PubMed]
- Jeon YS, Rhee YG. Factors associated with poor active anterior elevation after reverse total shoulder arthroplasty. J Shoulder Elbow Surg 2018;27:786-93. [Crossref] [PubMed]
- Berton A, Gulotta LV, Petrillo S, et al. The effect of humeral version on teres minor muscle moment arm, length, and impingement in reverse shoulder arthroplasty during activities of daily living. J Shoulder Elbow Surg 2015;24:578-86. [Crossref] [PubMed]
- Martin EJ, Duquin TR, Ehrensberger MT. Reverse total shoulder glenoid baseplate stability with superior glenoid bone loss. J Shoulder Elbow Surg 2017;26:1748-55. [Crossref] [PubMed]
- Denard PJ, Lederman E, Parsons BO, et al. Finite element analysis of glenoid-sided lateralization in reverse shoulder arthroplasty. J Orthop Res 2017;35:1548-55. [Crossref] [PubMed]
- Wong MT, Langohr GDG, Athwal GS, et al. Implant positioning in reverse shoulder arthroplasty has an impact on acromial stresses. J Shoulder Elbow Surg 2016;25:1889-95. [Crossref] [PubMed]
- Scalise J, Jaczynski A, Jacofsky M. The effect of glenosphere diameter and eccentricity on deltoid power in reverse shoulder arthroplasty. Bone Joint J 2016;98-B:218-23. [Crossref] [PubMed]
- de Boer FA, van Kampen PM, Huijsmans PE. The influence of subscapularis tendon reattachment on range of motion in reversed shoulder arthroplasty: A clinical study. Musculoskelet Surg 2016;100:121-6. [Crossref] [PubMed]
- Giles JW, Langohr GD, Johnson JA, et al. The rotator cuff muscles are antagonists after reverse total shoulder arthroplasty. J Shoulder Elbow Surg 2016;25:1592-600. [Crossref] [PubMed]
- Chan K, Langohr GDG, Mahaffy M, et al. Does Humeral Component Lateralization in Reverse Shoulder Arthroplasty Affect Rotator Cuff Torque? Evaluation in a Cadaver Model. Clin Orthop Relat Res 2017;475:2564-71. [Crossref] [PubMed]
Cite this article as: Hansen ML, Routman H. The biomechanics of current reverse shoulder replacement options. Ann Joint 2019;4:17.