Consideration of lateral augmentation in anatomic anterior cruciate ligament reconstruction
Introduction
The aim of surgical reconstruction of the anterior cruciate ligament (ACL) is to restore knee stability, allowing return to activity and prevention of secondary injury. Despite advances in modern arthroscopic techniques, return to high level sport has been unacceptably low in some studies (1) and significant rates of re-rupture and revision persist especially in the high-risk patient (2-5). Risk factors include young age, generalized ligamentous laxity, pivoting sport, high tibial slope, recurvatum and high-grade pivot (2,6-11). Anatomic intra-articular anterior cruciate ligament reconstruction (ACLR) techniques have been shown to reliably correct anteroposterior (translational) stability; however, they have failed to restore normal tibial rotational kinematics (12-14). Re-establishing rotational stability correlates with return to sport, functional scores, overall knee function and patient satisfaction (15,16).
Several structures in addition to the ACL have been identified as important contributors to rotational knee stability including the lateral meniscus, the medial meniscotibial ligament, and the anterolateral complex (ALC); comprising of the iliotibial band (ITB) and it’s Kaplan fibres, the capsulo-osseous layer of the ITB and the anterolateral capsule/anterolateral ligament (ALL) (17-21). The anterolateral capsulo-ligamentous structures have long been known to provide restraint to anterolateral rotation of the tibia and injuries in this region have been reported to occur at the time of ACL injury (22). For decades, surgeons have been aware that extra-articular augmentation procedures provide a powerful tool to control rotation of the knee and numerous techniques have been described (23-26). Historically these procedures were performed in isolation to address rotational stability. Over time they were largely abandoned due to concern of over-constraint of the knee and unsatisfactory clinical outcomes and the widespread adoption of arthroscopic intra-articular techniques (27-29).
Recent interest in the anatomical and biomechanical properties of the ALC has led to a resurgence in surgical techniques, specifically ALL reconstruction and lateral extra-articular tenodesis (LET), to address rotational stability at the time of ACL reconstruction.
Today, the concept of lateral augmentation procedures as an adjunct to anatomic intra-articular ACLR is being investigated with renewed optimism in the hopes of restoring rotational stability and improving clinical outcomes.
Anatomical and biomechanical rationale for the lateral augmentation procedures
The complex nature of the anterolateral structures of the knee that provide soft tissue restraint to abnormal internal rotation have been studied extensively both from an anatomical and biomechanical perspective. These structures include the ITB, ALL, anterolateral capsule and menisci. Non-standardised nomenclature and often conflicting anatomical descriptions have contributed to the ongoing confusion regarding the anatomy of the anterolateral knee and their individual contributions to rotary stability. A recent consensus meeting has helped to clarify many of these issues and is summarised (30).
Anatomy
The first description of an ALL like structure can be attributed to French surgeon Paul Segond who in 1879 described a “pearly, resistant, fibrous band inserting on the anterolateral aspect of the proximal tibia” (31). He showed this structure to be under tension with excessive internal rotation, which could result in an avulsion fracture with severe rotational stress. The “Segond” fracture is now considered pathognomonic of an ACL injury. The term “anterolateral ligament” was probably first introduced in 1962, in Kaplan’s original description of the iliotibial tract (32). In 1976 Hughston et al. identified that the ‘middle third of the lateral capsular ligament’ originated proximal to the lateral epicondyle and inserted distally to the tibial joint margin. They described this ligament as technically strong and a major lateral static support for the knee (33). They noted this structure to be torn in five acute clinical cases (four associated with ACL injury) and lax in 20 chronic cases (15 associated with ACL injury) when anterolateral rotational instability was evident. Müller in 1982 described the anatomy of the “anterolateral femorotibial ligament” and its role as a static rotational stabiliser (34). Feagin confirmed the findings of Hughston and Müller and provided the anatomical explanation for the Segond fracture (35). Vincent et al. performed an anatomical study during total knee arthroplasty from the intra-articular aspect of the joint and also described a ligamentous structure originating from the lateral femoral condyle and inserting into the lateral meniscus and anterolateral tibia (36).
In 2013, Claes et al. published their landmark anatomical study of the ALL, which received widespread publicity, including in the lay media (37). Since then, more than 100 scientific articles have been published on the ALL. Despite this extensive research, the presence, anatomy and function of the ALL remains controversial. A reason for this is the often-variable anatomy, particularly at the femoral origin and the different dissection protocols that have been used to isolate the ALL. An anatomic cadaveric study by Caterine et al. at our institution found the ALL could be identified in 100% of 19 specimens, and histologically the ALL was found to be a discrete ligamentous structure (20). Studies by Kennedy et al., Helito et al. and Vincent et al. also confirmed the presence of the ALL in 100% of analysed knees (36,38,39). In contrast to this, recent cadaveric studies by Runer et al. involving 44 specimens identified the ACL in 45% of knees and Herbst et al. only found a thickening of the middle third of the anterolateral capsule (consistent with the ALL) in 35% (7/20) of specimens (40,41). Multiple imaging studies have also analysed the presence and anatomy of the ALL. Rezansoff et al. performed a cadaveric study using tantulum beads and fluoroscopy to show variability of the femoral origin (42). In a retrospective review of 271 MRI studies, Claes et al found the ALL was clearly visible in 206 (76%) patients and injured in 162 (78.8%) patients with associated ACL injury (43). Hartigan et al. performed a retrospective MRI analysis of 72 knees and found the ALL to be visible in 100% of knees; however, inter- and intra-observer reliability between radiologists for detecting ALL tears was poor (44).
The most accepted anatomical landmarks for the ALL have recently been summarised by the ALC consensus group (30). The femoral origin is just proximal and posterior to the lateral femoral epicondyle as previously described by Dodds et al. and Kennedy et al. (38,45). The ALL then runs superficially over the proximal portion of the FCL and as it approaches the joint line, deeper fibres of the ligament provide attachment to the lateral meniscus. The insertion is midway between Gerdy’s tubercle and the fibula head on the proximal tibia just distal to the joint line (30) (Figure 1).
The ITB is made up of superficial, middle and deep layers. The deep layer occupies the most posterior segment of the ITB and blends with the superficial ITB distal to the lateral femoral epicondyle (41). The deep layer inserts just posterior to Gerdy’s tubercle on the anterolateral tibia together with the posterior fibers of the superficial ITB. The Kaplan fibers are distinct fiber bundles, which have a transverse course to the distal femoral metaphysis, from lateral to medial, to consistent bony prominences as described by Godin et al. (46). The Kaplan fibres have both proximal and distal segments and are in close proximity to the superior genicular artery (41). Terry et al. described the capsulo-osseous layer of the ITB, which is a triangular shaped structure on the deepest and most posterior portion of the ITB (47). The capsulo-osseous layer has fascial attachments to biceps femoris, and lateral gastrocnemius and its insertion point on the proximal tibia has been described as a distinct tubercle in studies by Herbst et al., Terry et al., and Godin et al. (41,46,47). A recent study by Albers also revealed its attachment to the Segond fragment, in addition to the ALL (48). Viera described the functional unit of the capsular-osseous layer, together with the ACL, as forming a spatial “horseshoe” form (49) (Figure 2).
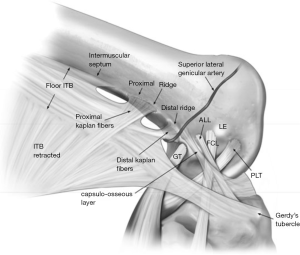
Biomechanics of the anterolateral structures
The ACL is the primary restraint to anterior translation of the knee and contributes to the restraint of varus/valgus laxity and internal tibial rotation. The ACL is comprised of two distinct fiber bundles, namely the anteromedial and posterolateral bundles; which tighten in flexion and extension, respectively. It has been found that the tibia can be translated anteriorly up to 30% more if it is free to rotate (50). This “coupled” rotation in response to anterior translation of the tibia results in a 3–10 degrees internal rotation when examined by hand that increases slightly with ACL rupture (50). The lateral knee compartment is inherently more mobile due to the convex bony geometry of the lateral tibial plateau and looser meniscocapsular attachments.
The most common mechanism leading to ACL injury involves a combination of non-contact forces similar to those described in the pivot shift test—axial loading of the knee with a valgus force as the knee moves from flexion to extension (51). This is reinforced by the fact that bony bruising identified on magnetic resonance imaging (MRI) of acute ACL injuries occurs at the posterolateral aspect of the tibia and anterolateral femoral condyle, which indicates this internal rotation movement (52). The combination of forces leading to “isolated” ACL ruptures often results in additional injuries to the lateral soft tissue capsuloligamentous structures that are increasingly being recognized.
There have been multiple structures on the lateral side of the knee that have been identified through past studies as having an important role in restraining anterolateral rotatory laxity (17-21). More recent research has focused on the influence of the ALL in the ACL-deficient knee (20,21,38,41,45,53,54). However, many studies have provided inconsistent anatomical descriptions for the ALL. This heterogeneity has led to conflicting results, and possibly under or over-estimation of the contribution of each structure to knee biomechanics. Many studies also removed the ITB prior to testing of the deeper anterolateral structures; therefore, non-physiologic patterns of rotary instability are generated after disruption to the dominant functional unit. Kennedy found the ALL to have a mean tensile strength of 175 N and a mean stiffness of 20 N/mm (38). In 12 specimens, they found four different mechanisms of failure; ligamentous tear at the femoral origin in four specimens, in the mid substance in four, at the tibial insertion in one and by a bony avulsion, i.e., Ségond fracture, in six. However, it should be noted that the line of pull in these experiments was non-physiologic. In contrast, Zens et al. found the ALL had an ultimate tensile strength of only 50±15 N, overall stiffness of 4.2 N/mm and a small cross-sectional area of only 1.54 mm2 (54). The ALL failed at mid-substance in all specimens and did not induce a bone avulsion. Dodds et al. found the ALL to be isometric from extension to early flexion (0–60°), and lengthened with internal tibial rotation, strongly supporting a role in rotational control (45). Two studies utilising a navigation system and manually applied forces by Monaco et al. (55) and Spencer et al. (56) investigated the effect of sectioning the ACL and lateral capsular ligament/ALL. Monaco et al demonstrated a maximal increase in internal rotation of 5.5 degrees at 30 degrees flexion while Spencer et al found an increase of 2 degrees in extension during a dynamic pivot shift test. Lording et al. performed a cadaveric study on the ACL intact knee using a robotic knee examination device, and demonstrated that dividing the ALL increased internal rotation by 2.4° at 30° flexion (57). Rasmussen et al. used a six degree of freedom robot to show that sectioning the ALL resulted in a maximum increase of internal rotation of 3.3 degrees at 45 degrees of knee flexion in a ACL deficient knee (58). Sonnery-Cottet et al. reported an increase in coupled internal rotation during the simulated pivot-shift after section of the ALL in an ACL deficient knee by 6 degrees; however further sectioning of the ITB increased coupled rotation by an additional 9 degrees (59). Parsons et al., using robotic technology, found the ALL to be the primary restraint to internal rotation at knee flexion angles greater than 35°, with the ACL conversely providing the most restraint nearing extension (21). However, the contribution of the ALL was likely over-estimated given the ITB was removed prior to testing. In contrast to previous studies, Kittl et al. and Huser et al. concluded the ALL did not have a significant role in internal rotational control (53,60).
Despite the current interest in the ALL, recent biomechanical studies suggest that the ITB and its attachment to the distal femur via Kaplan’s fibres, provides the dominant restraint to internal rotation of the tibia (53). The most isometric structure of the anterolateral complex relating to rotational control is also the deep and posterior layers of the ITB via its Kaplan fibres (61). The study by Kittl et al. measured the individual reductions in tibial internal rotation torque after serial sectioning of the anterolateral structures: the superficial ITB, the deep/capsule-osseous fibres of the ITB; the ALL, then the anterolateral joint capsule (53). This was performed in both ACL deficient and ACL intact knees. The main conclusion of this study was that the ITB was the primary restraint to tibial internal rotation. The superficial ITB significantly restrained internal rotation at high flexion angles while the deep ITB was more responsible at lower flexion angles. The ACL only provided significant rotational control in full knee extension. During a simulated pivot-shift test in the ACL-deficient group, the ITB was found to provide 72%±14% of the restraint at 45° flexion. The ALL and other anterolateral structures made only a minor contribution in restraining the pivot shift. They concluded the ALL was relatively weak and poorly aligned to resist internal rotation. These results from Kittl et al. are in line with the operative observations of Terry et al., who described injury to the deep capsulo-osseous layer of the ITB in 93% of the functionally unstable knees they reconstructed, and this damage correlated significantly with higher grades of pivot shift (47). Older studies by Fetto and Jakob also describe the dominant contribution of the ITB to the pivot-shift maneuver (62,63). In contrast to the ALL, Noyes also found an 18-mm-wide strip of the ITB to have a much greater mean tensile strength of 769N (64).
Lateral meniscal deficiency and isolated posterior root tears have also been found to contribute to rotatory instability in the ACL-deficient knee (17,22). Musahl et al. demonstrated that lateral meniscal loss had a significant role in the manifestation of the pivot shift. A recent study at our facility also suggested the lateral meniscus has a role in controlling internal rotation in lower flexion angles, while also having an intimate relationship with the ALL insertion on the tibia (20,65). Peltier et al. and Dephillpo et al. have also demonstrated the medial meniscotibial ligament’s importance as a restraint to rotatory laxity (18,66).
Lateral augmentation procedures
Lateral extra-articular procedures were developed to restrain anterior translation and rotation in the ACL deficient knee, prior to the era of intra-articular ACLR procedures (67). Extra-articular procedures have a biomechanical advantage over intra-articular reconstruction with regards to rotational control, as the longer lever arm exerted by a peripherally-based graft is theoretically more able to resist torque. Ellison [1978] described the ACL as “the hub of the wheel” and suggested that “it is easier to control rotation of a wheel at its rim than at its hub” (68).
There are numerous LET techniques described and all are inherently non-anatomic in design. The more commonly used techniques in the past have included the Lemaire technique, modified Lemaire procedure, MacIntosh lateral substitution reconstruction, Losee technique, and Ellison’s distal Iliotibial Tract transfer (23-26). In the majority of techniques, a strip of the ITB with variable length was mobilised and tunnelled either under or over the FCL and anchored at differing locations on the lateral femoral condyle then fixed back to the proximal tibia (Figure 3).
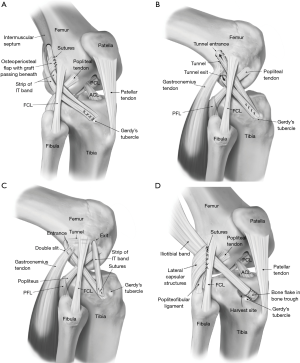
Results for isolated LET procedures have been generally poor. Return to their pre-injury level of sporting activity was seen in less than half of the patients with a MacIntosh procedure, despite abolishment of a positive pivot shift in 84% of patients (28). LET techniques were found to result in lateral compartment over constraint of motion, an externally rotated resting tibial position and premature development of osteoarthritis (69-71). Tensioning of LET grafts in excessive external rotation and prolonged immobilisation, as described in past techniques, most likely contributed to these unsatisfactory outcomes. Although over constraint has the theoretical potential to increase the lateral compartment joint reaction forces and hence increase the risk of osteoarthritis, currently there is no clear evidence of increased lateral compartment osteoarthritis in the literature, when intra-articular ACLR is augmented with a LET (72-74).
ALL reconstruction techniques have been developed almost exclusively over the last 5 years since the publication of Claes’ landmark paper. Described techniques include those by Sonnery-Cottet et al. and Helito et al. (75,76), which use a continuous graft construct extending from the intra-articular ACL component; while other techniques by Smith et al. and Chahla et al. (77,78) use a free gracilis graft and semitendinosis allograft respectively (Figure 4).
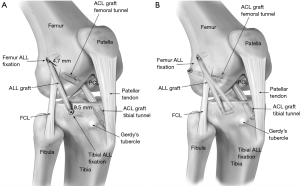
Sonnery-Cottet utilises a three-strand semitendinosus graft coupled to a free single-strand gracilis graft (75). This results in a quadrupled ACL graft, tailing into a single strand of gracilis, for the ALL reconstruction. They identify a femoral point slightly posterior and proximal to the lateral femoral epicondyle corresponding to the ALL origin. This location is drilled using an outside in technique that serves both as the femoral attachment for the ALL reconstruction and the femoral tunnel for the intra-articular ACLR. After the ACL component of the graft is tunnelled, the single gracilis strand exiting from the femoral tunnel is used to complete the ALL reconstruction. The gracilis graft is tunnelled underneath the ITB and passed through an osseous tibial tunnel from posterior to anterior, then rerouted onto itself for fixation back at the femoral origin. This distal double limb technique creates an inverted Y shaped reconstruction that differs from most other described ALL reconstruction techniques, which generally use a single fixation point on the tibia. It is possible that the two bundles spread apart at the tibia, provides for differential engagement of individual bundles, resulting in net isometricity and better rotational control at varying flexion angles, making it a biomechanically superior construct compared to a single bundle ALL reconstruction.
Biomechanical studies of combined ACLR + lateral augmentation procedures
When combined with ACLR, lateral augmentation procedures have demonstrated consistent ability to restore rotational stability (73). Most experimental “time zero” studies favour the use of LET over anatomic ALL reconstruction (56,79,80).
LET has been demonstrated to work synergistically to protect the intra-articular ACL graft. Modern anatomic ACLR techniques place the graft at a more oblique angle that theoretically exposes the graft to higher than normal forces since it should in theory resist more rotational torques (81). This may lead to graft failure due to stretching or rupture. The addition of a LET has been shown to reduce the stress on an ACL graft by 43% in a cadaver model (82). Another cadaveric study demonstrated a load-sharing relationship between the LET and an intra-articular graft during both anterior translation and internal rotation (70). Monaco et al. performed an in vivo study and found at the time of reconstruction that adding an LET to a single bundle ACLR more significantly reduced tibial internal rotation compared to both single and double bundle ACLR alone (83). Zaffagnini also showed augmenting with a LET provided improved laxity reduction in varus/valgus stress test at full extension and better restraint to internal tibial rotation at 90 degrees flexion (84).
Spencer et al. performed a biomechanical analysis after sectioning and then reconstruction of the ALL using optical tracking and manually applied forces (56) in twelve cadaveric knees. Reconstruction of the ALL, based on the anatomic landmarks of Claes, failed to restore the kinematics of the intact native ALL. In contrast, an LET performed using a modified Lemaire technique (with the ITB routed deep to the FCL and attached to the femoral metaphysis) resulted in a significant reduction in both anterior translation and internal rotation in the ACL-deficient state. They hypothesised that when the ITB graft is routed underneath the FCL, the FCL acts as a pulley to maintain relative isometry, whereas the ALL, with a distal and anterior origin, becomes more lax nearing extension and therefore is ineffective in controlling the pivot shift. They noticed the tendency of an ALL reconstruction to over constrain the lateral compartment, which is also a concern that has been raised from the study by Schon et al. (79). The latter authors concluded that anatomic ALL reconstruction was not capable of restoring anterolateral stability without introducing significant over constraint of the knee at any graft fixation angle. However, it must be noted that Schon et al. used a high graft tension of 88 N in their study. A cadaveric study by Geeslin et al. compared ALL reconstruction (based on the anatomy described by Kennedy et al. (38) to the modified Lemaire LET, utilising multiple different knee flexion angles and graft tension parameters at fixation (85). The modified Lemaire LET resulted in significantly greater reduction in laxity with internal rotation and pivot shift testing than the ALL reconstruction; however, both reconstructions caused an element of over-constraint.
Inderhaug et al. performed a cadaveric study utilising a six degrees of freedom rig and optical tracking to compare two different LET techniques; the modified Lemaire (passed both superficial and deep to FCL) and the MacIntosh tenodesis with an ALL reconstruction, tensioned at both 20 and 40 N (80). The ALL reconstruction utilised a free gracilis graft that was fixed proximal and posterior to the lateral epicondyle, in keeping with the previously discussed accepted anatomical landmark. The authors found that with 20 N of graft tension, the MacIntosh and Lemaire (deep to the FCL) procedures restored anterior translation and rotational kinematics to the intact state, whereas the ALL reconstruction had persistent increased rotation. The superficial Lemaire procedure, where the graft was passed over the FCL, lead to over constraint and therefore provided the least favourable kinematic effects. These findings led the authors to infer the ‘‘pulley effect’’ of the FCL helped provide more consistent graft behaviour by retaining the graft posterior to the axis of rotation throughout the range of knee motion, even with differing femoral fixation sites. This conclusion of Inderhaug et al. is in line with that of Kittl et al. who found that a graft attached proximal to the lateral femoral epicondyle and running underneath the FCL provided the most desirable graft behaviour, maintaining more isometry throughout knee range of motion (53). Another biomechanical advantage of the LET procedure, especially if passed under FCL, is its more oblique course across the joint when compared with the perpendicular (vertical) alignment of an anatomic ALL reconstruction. By the pulley action of the FCL and lateral epicondyle a graft orientation more parallel to the joint is maintained through most of the flexion range. This, coupled with the more anterior attachment of a tenodesis based on Gerdy’s tubercle in comparison to the ALL tibial attachment, creates a more efficient orientation to restrain tibial internal rotation (50).
Clinical evidence for combined ACLR & lateral augmentation procedures
At present, there is no high-level evidence to define clear indications for the addition of lateral augmentation procedures to an ACLR. Interpretation of previous studies has been challenging due to the significant heterogeneity of inclusion and exclusion criteria, surgical techniques and often small numbers. There are currently no clinical trials that have directly compared the results of LET procedures to ALL reconstructions in augmenting ACLR.
Systematic reviews with meta-analyses comparing the addition of a lateral augmentation procedure to an ACLR have universally demonstrated improved rotational laxity control but have failed to show an impact on patient-reported outcomes (72,73,86,87). Rezende et al. compared randomized controlled trials of isolated ACLR versus combined ACL reconstruction (86). Combined procedures were found to improve rotational and anteroposterior stability with no significant difference in failure rates or patient reported outcome measures. Meta-analyses by Hewison et al. and Song et al. also revealed a statistically significant reduction of the pivot shift after ACLR with LET, but no significant advantage in terms of anterior translation or IKDC scores (73,87). Devitt et al. performed a systematic review to assess whether the addition of LET provides greater control of rotational laxity and improves clinical outcomes compared with ACLR alone and to assess the impact of early (<12 months) versus delayed reconstruction (72). They found a significant reduction in postoperative pivot shift only in the delayed reconstruction group and no effect on clinical, and functional outcomes in either the early or delayed groups. Williams et al. performed a case-control study of isolated ACLR (48 patients) versus combined ACLR + modified Lemaire LET (49 patients) with short term clinical examination results favoring the combined group for improved rotational stability (88). They found a reduced incidence of pivot glide 9% vs. 2% and also greater AP stability nearing extension (Grade 1 Lachman 26% vs. 6%); however, a higher percentage of knees had a grade 1 anterior draw 10% vs. 19% respectively.
Currently, the best clinical evidence for the addition of a lateral augmentation procedure comes from the Scientific ACL Network International (SANTI) group. They have performed a prospective comparative study of 502 patients undergoing ACLR stratified into 3 different treatment groups with minimum two year follow up (89). They demonstrated significantly lower graft failure rates with combined hamstring + ALL reconstruction, with a hazard ratio of 2.5 times less than isolated bone patellar tendon bone grafts and 3.1 times less than isolated hamstring tendon grafts. The combined hamstring +ALL reconstruction group also had a significantly higher rate of return to pre-injury level sport when compared with isolated hamstring grafts (odds ratio 1.938). In a later study of 548 patients with combined hamstring ACL + ALL reconstructions they looked at reoperation rates at 35.5±8.0 months (range, 24–54 months) (90). Repeat operation for graft failure was low at 2.6% and there were only 3 patients who had a complication specific to the ALL reconstruction which were all related to the femoral hardware (all 3 underwent removal). In a third cohort study of 383 patients, the SANTI group also showed a protective effect of the ALL reconstruction after meniscal repair at the time of ACLR with a survival of 91.2% vs. 83.8% at 36 months (91).
At our centre, we are currently leading a randomized multi-centre clinical trial (Clinical Trials.gov NCT02018354) comparing ACLR with or without LET augmentation in approximately 600 patients who are deemed at high risk of graft failure. Patient inclusion criteria is age under 25 and two or more of the following characteristics: participation in a pivoting sport, greater than a grade 2 pivot shift, or generalized ligamentous laxity. The results will be available in 2019 when we hope to be able to further define the ideal indications for the addition of an LET to primary ACL reconstruction.
In regard to complications, the concern regarding over constraint and the development of osteoarthritis appears unfounded. A recent systematic review by Devitt et al. failed to show evidence of increased prevalence of osteoarthritis (OA) following combined ACLR + LET procedures (72). Long term outcome studies by Pernin et al. and Zaffagini et al., with greater than 20 years follow up, also failed to demonstrate increased risk of lateral compartment OA (74,92). From our own experience, early surgical complications related to the LET are rare. We have infrequently encountered postoperative hematoma of the lateral aspect of the knee which we feel can be prevented by careful use of electrocautery during identification and preparation of the femoral attachment site of the IT band graft. Less than 1% of patients in our series have required removal of symptomatic hardware from the femur.
The Fowler Kennedy approach
Our preferred lateral augmentation technique is the modified Lemaire LET procedure rather than an ALL reconstruction. This is based upon the superior biomechanical evidence for this procedure over the ALL reconstruction, and the long clinical track record that is associated with this procedure.
Indications
As yet there exists no high-level prospective evidence to guide the use of LET during primary ACL reconstruction. Based on expert opinion, the patient most likely to benefit from primary LET may have one or more of young age (<25 years), high grade rotational laxity (grade 2–3 pivot shift or >5 mm lateral compartment translation), generalized ligamentous laxity (knee hyperextension >10 degrees), elevated tibial posterior slope, meniscal deficiency, magnetic resonance imaging (MRI) evidence of anterolateral capsule injury or participation in pivoting sport (30). At our centre, we strongly consider performing LET during primary ACL procedures on patients with a grade three pivot shift and generalized ligamentous laxity, with additional consideration given to patients wishing to return to pivoting sport. We routinely perform LET during revision ACL reconstruction when the knee displays no other rotational (i.e., posterolateral) laxity.
Surgical technique (Figure 5)
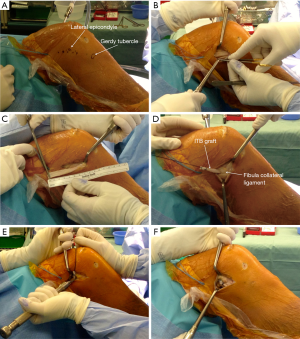
Following completion of the intra-articular ACL reconstruction, the knee is placed at 90 degrees of flexion and a 6 cm longitudinal incision is made approximately 1 cm posterior to the lateral femoral epicondyle. Subcutaneous tissue is divided sharply down to the level of the ITB and fat is swept off the ITB posteriorly with a gauze sponge to identify its posterior margin. Ensuring the posterior fibres of the ITB are undisturbed (so as not to damage the deep capsule-osseous layer), we harvest an 8 cm long by 1 cm wide strip of ITB that is released along its entire length, including any deep attachments, but left attached distally at the Gerdy’s tubercle insertion. The proximal 2 cm of the ITB graft are then whip stitched with #1 Vicryl suture.
The fibular collateral ligament (FCL) is then identified using palpation, facilitated by placing the knee in figure-of-four position. With a #15 scalpel, small capsular incisions are created just anterior and posterior to the proximal aspect of the FCL and Metzenbaum scissors are passed deep to the FCL taking care to remain extra-capsular and prevent damage to the popliteus tendon. A Fraser clamp is then passed deep to the FCL and the ITB graft is brought under FCL from distal to proximal using the Fraser clamp.
The femoral attachment site of the tenodesis is just proximal and posterior to the FCL origin, just anterior to the attachment site of the distal Kaplan fibres of the ITB and in close proximity to the superior lateral genicular artery. The periosteum is removed with a Cobb elevator on the metaphyseal flare of the lateral femoral condyle. The knee is then placed in 60–70 degrees of flexion with the tibia and foot in neutral rotation to avoid over-constraining the lateral joint compartment and restricting rotational freedom. The graft is held taut with minimal tension (<20 N) and secured to the femur with a Richards’ staple (Smith and Nephew Inc, Andover, MA). Excess graft length is then folded and sutured back onto itself using a free needle on the #1 Vicryl whip stitch.
Weight bearing and range of motion is allowed as tolerated immediately, with the ACL reconstruction and meniscal pathology dictating rehabilitation.
Pearls and pitfalls
- Use Metzenbaum scissors to dissect the deep plane of the ITB graft proximally first as this plane is more difficult to identify distally.
- The knee can be placed into figure four position to place the FCL on stretch and aid its identification by palpation.
- At the femoral attachment site of the tenodesis, there is a small fat pad in the area proximal and lateral to the lateral gastrocnemius tendon. This fat pad should be cleared down to femur with electrocautery as the superolateral geniculate artery is in close proximity as well as small veins that are usually present within it. These vessels are coagulated if required on a case by case basis.
- If suspensory loop femoral fixation is used for the ACL graft, the button is typically in the area of femoral LET graft attachment, and care should be taken to avoid damaging the button.
- The tenodesis can be thought of as a check-rein and as such, minimal tension is placed on the LET graft during femoral fixation with the knee placed at 60 degrees of flexion and the foot in neutral rotation to avoid over-constraint.
Conclusions
Recent anatomic and biomechanical research has comprehensively described the anterolateral soft tissue structures and their contribution to controlling rotational stability. The literature supports the biomechanical benefits of the addition of lateral augmentation procedures to ACLR with the ability to reduce internal rotation laxity and control the pivot shift. Our preferred augmentation technique is a LET for reasons cited above. With modern techniques, it is a low morbidity procedure with minimal complications. More clinical and experimental studies are needed to evaluate the long-term clinical outcomes and define the indications for the procedure.
Acknowledgments
We would like to acknowledge the following team member [Stacey Wanlin (Orthopaedic Sport Medicine Research Manager & Fellowship Coordinator, Fowler Kennedy Sport Medicine Clinic, 3M Centre, Western University, 1151 Richmond Street, London, ON N6A 3K7)] for her contribution to organising figure permissions.
Funding: None.
Footnote
Provenance and Peer Review: This article was commissioned by the Guest Editor (Takeshi Muneta) for the series “Anatomic Reconstruction of Anterior Cruciate Ligament - Concept, Indication, and Its Efficacy” published in Annals of Joint. The article has undergone external peer review.
Conflict of Interest: The series “Anatomic Reconstruction of Anterior Cruciate Ligament - Concept, Indication, and Its Efficacy” was commissioned by the editorial office without any funding or sponsorship. AG is a paid consultant for Smith and Nephew Inc. The authors have no other conflicts of interest to declare.
Ethical Statement: The authors are accountable for all aspects of the work in ensuring that questions related to the accuracy or integrity of any part of the work are appropriately investigated and resolved.
Open Access Statement: This is an Open Access article distributed in accordance with the Creative Commons Attribution-NonCommercial-NoDerivs 4.0 International License (CC BY-NC-ND 4.0), which permits the non-commercial replication and distribution of the article with the strict proviso that no changes or edits are made and the original work is properly cited (including links to both the formal publication through the relevant DOI and the license). See: https://creativecommons.org/licenses/by-nc-nd/4.0/.
References
- Ardern CL, Webster KE, Taylor NF, et al. Return to sport following anterior cruciate ligament reconstruction surgery: a systematic review and meta-analysis of the state of play. Br J Sports Med 2011;45:596-606. [Crossref] [PubMed]
- Hettrich CM, Dunn WR, Reinke EK, et al. The Rate of Subsequent Surgery and Predictors After Anterior Cruciate Ligament Reconstruction: Two- and 6-Year Follow-up Results From a Multicenter Cohort. Am J Sports Med 2013;41:1534-40. [Crossref] [PubMed]
- Mariscalco MW, Flanigan DC, Mitchell J, et al. The influence of hamstring autograft size on patient-reported outcomes and risk of revision after anterior cruciate ligament reconstruction: a Multicenter Orthopaedic Outcomes Network (MOON) Cohort Study. Arthroscopy 2013;29:1948-53. [Crossref] [PubMed]
- Shelbourne KD, Gray T, Haro M. Incidence of Subsequent Injury to Either Knee Within 5 Years After Anterior Cruciate Ligament Reconstruction With Patellar Tendon Autograft. Am J Sports Med 2009;37:246-51. [Crossref] [PubMed]
- Wright RW, Magnussen RA, Dunn WR, et al. Ipsilateral Graft and Contralateral ACL Rupture at Five Years or More Following ACL Reconstruction. J Bone Joint Surg Am 2011;93:1159-65. [Crossref] [PubMed]
- Webster KE, Feller JA, Leigh WB, et al. Younger Patients Are at Increased Risk for Graft Rupture and Contralateral Injury After Anterior Cruciate Ligament Reconstruction. Am J Sports Med 2014;42:641-7. [Crossref] [PubMed]
- Akhtar MA, Bhattacharya R, Keating JF. Generalized ligamentous laxity and revision ACL surgery: is there a relation? Knee 2016;23:1148-53. [Crossref] [PubMed]
- Salmon L, Russell V, Musgrove T, et al. Incidence and risk factors for graft rupture and contralateral rupture after anterior cruciate ligament reconstruction Arthroscopy 2005;21:948-57. [Crossref] [PubMed]
- Kaeding CC, Pedroza AD, Reinke EK, et al. Risk Factors and Predictors of Subsequent ACL Injury in either Knee after ACL Reconstruction: Prospective Analysis of 2488 Primary ACL Reconstructions from the MOON Cohort Am J Sports Med 2015;43:1583-90. [Crossref] [PubMed]
- Salmon LJ, Heath E, Akrawi H, et al. 20-Year Outcomes of Anterior Cruciate Ligament Reconstruction With Hamstring Tendon Autograft: The Catastrophic Effect of Age and Posterior Tibial Slope. Am J Sports Med 2018;46:531-43. [Crossref] [PubMed]
- Magnussen RA, Reinke EK, Huston LJ, et al. Effect of High-Grade Preoperative Knee Laxity on Anterior Cruciate Ligament Reconstruction Outcomes. Am J Sports Med 2016;44:3077-82. [Crossref] [PubMed]
- Woo SLY, Kanamori A, Zeminski J, et al. The effectiveness of reconstruction of the anterior cruciate ligament with hamstrings and patellar tendon. A cadaveric study comparing anterior tibial and rotational loads. J Bone Joint Surg Am 2002;84-A:907-14. [Crossref] [PubMed]
- Ristanis S, Stergiou N, Patras K, et al. Excessive Tibial Rotation During High-Demand Activities Is Not Restored by Anterior Cruciate Ligament Reconstruction. Arthroscopy 2005;21:1323-9. [Crossref] [PubMed]
- Georgoulis AD, Ristanis S, Chouliaras V, et al. Tibial Rotation is Not Restored after ACL Reconstruction with a Hamstring Graft. Clin Orthop Relat Res 2007;89-94. [Crossref] [PubMed]
- Ayeni OR, Chahal M, Tran MN, et al. Pivot shift as an outcome measure for ACL reconstruction: a systematic review. Knee Surg Sports Traumatol Arthrosc 2012;20:767-77. [Crossref] [PubMed]
- Kocher MS. Relationships Between Objective Assessment of Ligament Stability and Subjective Assessment of Symptoms and Function After Anterior Cruciate Ligament Reconstruction. Am J Sports Med 2004;32:629-34. [Crossref] [PubMed]
- Musahl V, Citak M, O'Loughlin PF, et al. The Effect of Medial Versus Lateral Meniscectomy on the Stability of the Anterior Cruciate Ligament-Deficient Knee. Am J Sports Med 2010;38:1591-7. [Crossref] [PubMed]
- Peltier A, Lording T, Maubisson L, et al. The role of the meniscotibial ligament in posteromedial rotational knee stability. Knee Surg Sports Traumatol Arthrosc 2015;23:2967-73. [Crossref] [PubMed]
- Vieira ELC, Vieira EÁ, da Silva RT, et al. An anatomic study of the iliotibial tract. Arthroscopy 2007;23:269-74. [Crossref] [PubMed]
- Caterine S, Litchfield R, Johnson M, et al. A cadaveric study of the anterolateral ligament: re-introducing the lateral capsular ligament. Knee Surg Sports Traumatol Arthrosc 2015;23:3186-95. [Crossref] [PubMed]
- Parsons EM, Gee AO, Spiekerman C, et al. The biomechanical function of the anterolateral ligament of the knee. Am J Sports Med 2015;43:669-74. [Crossref] [PubMed]
- Van Dyck P, Clockaerts S, Vanhoenacker FM, et al. Anterolateral ligament abnormalities in patients with acute anterior cruciate ligament rupture are associated with lateral meniscal and osseous injuries. Eur Radiol 2016;26:3383-91. [Crossref] [PubMed]
- Lemaire M. Instabilité chronique du genou. Techniques et résultats des plasties ligamentaires en traumatologie sportive. J Chir 1975;110:281-94.
- MacIntosh DL. Lateral Substitution Reconstruction. J Bone Joint Surg Br 1976;58-B:142.
- Ellison AE. Distal iliotibial-band transfer for anterolateral rotatory instability of the knee. J Bone Joint Surg Am 1979;61:330-7. [Crossref] [PubMed]
- Losee RE, Johnson TR, Southwick WO. Anterior subluxation of the lateral tibial plateau. A diagnostic test and operative repair. J Bone Joint Surg Am 1978;60:1015-30. [Crossref] [PubMed]
- Neyret P, Palomo JR, Donell ST, et al. Extra-articular tenodesis for anterior cruciate ligament rupture in amateur skiers. Br J Sports Med 1994;28:31-4. [Crossref] [PubMed]
- Ireland J, Trickey EL. Macintosh tenodesis for anterolateral instability of the knee. J Bone Joint Surg Br 1980;62:340-5. [Crossref] [PubMed]
- Amirault JD, Cameron JC, MacIntosh DL, et al. Chronic anterior cruciate ligament deficiency. Long-term results of MacIntosh's lateral substitution reconstruction. J Bone Joint Surg Br 1988;70:622-4. [Crossref] [PubMed]
- Getgood A, Brown C, Lording T, et al. The anterolateral complex of the knee: results from the International ALC Consensus Group Meeting. Knee Surg Sports Traumatol Arthrosc 2018; [Epub ahead of print]. [Crossref] [PubMed]
- Segond P. Recherches cliniques et experimentales sur les epanchements sanguins du genou par entorse. Paris: Progres Medical; 1879.
- Kaplan EB. The iliotibial tract; clinical and morphological significance. J Bone Joint Surg Am 1958;40-A:817-32. [Crossref] [PubMed]
- Hughston JC, Andrews JR, Cross MJ, et al. Classification of knee ligament instabilities. Part II. The lateral compartment. J Bone Joint Surg Am 1976;58:173-9. [Crossref] [PubMed]
- Müller W. Functional anatomy and clinical findings of the knee joint. Helv Chir Acta 1984;51:505-14. [PubMed]
- Feagin JA. The crucial ligaments: diagnosis and treatment of ligamentous injuries about the knee. New York: Churchill Livingstone, 1988.
- Vincent JP, Magnussen RA, Gezmez F, et al. The anterolateral ligament of the human knee: an anatomicand histologic study. Knee Surg Sports Traumatol Arthrosc 2012;20:147-52. [Crossref] [PubMed]
- Claes S, Vereecke E, Maes M, et al. Anatomy of the anterolateral ligament of the knee. J Anat 2013;223:321-8. [Crossref] [PubMed]
- Kennedy MI, Claes S, Fuso FA, et al. The anterolateral ligament: an anatomic, radiographic, and biomechanical analysis. Am J Sports Med 2015;43:1606-15. [Crossref] [PubMed]
- Helito CP, Demange MK, Bonadio MB, et al. Anatomy and histology of the knee anterolateral ligament. Orthop J Sports Med 2013;1:2325967113513546 [Crossref] [PubMed]
- Runer A, Birkmaier S, Pamminger M, et al. The anterolateral ligament of the knee: A dissection study. Knee 2016;23:8-12. [Crossref] [PubMed]
- Herbst E, Albers M, Burnham JM, et al. The Anterolateral Complex of the Knee. Orthop J Sports Med 2017;5:2325967117730805 [Crossref] [PubMed]
- Rezansoff AJ, Caterine S, Spencer L, et al. Radiographic landmarks for surgical reconstruction of the anterolateral ligament of the knee. Knee Surg Sports Traumatol Arthrosc 2015;23:3196-201. [Crossref] [PubMed]
- Claes S, Bartholomeeusen S, Bellemans J. High prevalence of anterolateral ligament abnormalities in magnetic resonance images of anterior cruciate ligament-injured knees. Acta Orthop Belg 2014;80:45-9. [PubMed]
- Hartigan DE, Carroll KW, Kosarek FJ, et al. Visibility of anterolateral ligament tears in anterior cruciate ligament-deficient knees with standard 1.5-Tesla magnetic resonance imaging. Arthroscopy 2016;32:2061-5. [Crossref] [PubMed]
- Dodds AL, Halewood C, Gupte CM, et al. The anterolateral ligament: Anatomy, length changes and association with the Segond fracture. Bone Joint J 2014;96-B:325-31. [Crossref] [PubMed]
- Godin JA, Chahla J, Moatshe G, et al. A Comprehensive Reanalysis of the Distal Iliotibial Band: Quantitative Anatomy, Radiographic Markers, and Biomechanical Properties. Am J Sports Med 2017;45:2595-603. [Crossref] [PubMed]
- Terry GC, Hughston JC, Norwood LA. The anatomy of the iliopatellar band and iliotibial tract. Am J Sports Med 1986;14:39-45. [Crossref] [PubMed]
- Albers M, Shaikh H, Herbst E, et al. The iliotibial band and anterolateral capsule have a combined attachment to the Segond fracture. Knee Surg Sports Traumatol Arthrosc 2018;26:1305-10. [Crossref] [PubMed]
- Vieira EL, Vieira EA, da Silva RT, et al. An anatomic study of the iliotibial tract. Arthroscopy 2007;23:269-74. [Crossref] [PubMed]
- Amis AA. Anterolateral knee biomechanics. Knee Surg Sports Traumatol Arthrosc 2017;25:1015-23. [Crossref] [PubMed]
- Boden BP, Sheehan FT, Torg JS, et al. Noncontact Anterior Cruciate Ligament Injuries: Mechanisms and Risk Factors. J Am Acad Orthop Surg 2010;18:520-7. [Crossref] [PubMed]
- Song GY, Zhang H, Wang QQ, et al. Bone contusions after acute noncontact anterior cruciate ligament injury are associated with knee joint laxity, concomitant meniscal lesions, and anterolateral ligament abnormality. Arthroscopy 2016;32:2331-41. [Crossref] [PubMed]
- Kittl C, Halewood C, Stephen JM, et al. Length change patterns in the lateral extra-articular structures of the knee and related reconstructions. Am J Sports Med 2015;43:354-62. [Crossref] [PubMed]
- Zens M, Feucht MJ, Ruhhammer J, et al. Mechanical tensile properties of the anterolateral ligament. J Exp Orthop 2015;2:7. [Crossref] [PubMed]
- Monaco E, Ferretti A, Labianca L, et al. Navigated knee kinematics after cutting of the ACL and its secondary restraint. Knee Surg Sports Traumatol Arthrosc 2012;20:870-7. [Crossref] [PubMed]
- Spencer L, Burkhart TA, Tran MN, et al. Biomechanical Analysis of Simulated Clinical Testing and Reconstruction of the Anterolateral Ligament of the Knee. Am J Sports Med 2015;43:2189-97. [Crossref] [PubMed]
- Lording T, Stinton SK, Neyret P, et al. Diagnostic findings caused by cutting of the iliotibial tract and anterolateral ligament in an ACL intact knee using a standardized and automated clinical knee examination. Knee Surg Sports Traumatol Arthrosc 2017;25:1161-9. [Crossref] [PubMed]
- Rasmussen MT, Nitri M, Williams BT, et al. An in vitro robotic assessment of the anterolateral ligament in the setting of an anterior cruciate ligament injury. Am J Sports Med 2016;44:585-92. [Crossref] [PubMed]
- Sonnery-Cottet B, Lutz C, Daggett M, et al. The involvement of the anterolateral ligament in rotational control of the knee. Am J Sports Med 2016;44:1209-14. [Crossref] [PubMed]
- Huser LE, Noyes FR, Jurgensmeier D, et al. Anterolateral Ligament and Iliotibial Band Control of Rotational Stability in the Anterior Cruciate Ligament-Intact Knee: Defined by Tibiofemoral Compartment Translations and Rotations. Arthroscopy 2017;33:595-604. [Crossref] [PubMed]
- Kittl C, Halewood C, Stephen JM, et al. Length change patterns in the lateral extra-articular structures of the knee and related reconstructions. Am J Sports Med 2015;43:354-62. [Crossref] [PubMed]
- Fetto JF, Marshall JL. Injury to the anterior cruciate ligament producing the pivot-shift sign. J Bone Joint Surg Am 1979;61:710-4. [Crossref] [PubMed]
- Jakob RP, Hassler H, Staeubli HU. Observations on rotatory instability of the lateral compartment of the knee. Experimental studies on the functional anatomy and the pathomechanism of the true and the reversed pivot shift sign. Acta Orthop Scand Suppl 1981;191:1-32. [Crossref] [PubMed]
- Noyes FR, Butler DL, Grood ES, et al. Biomechanical analysis of human ligament grafts used in knee ligament repairs and reconstructions. J Bone Joint Surg (Am) 1984;66:344-52. [Crossref] [PubMed]
- Lording T, Corbo G, Bryant D, et al. Rotational Laxity Control by the Anterolateral Ligament and the Lateral Meniscus Is Dependent on Knee Flexion Angle: A Cadaveric Biomechanical Study. Clin Orthop Relat Res 2017;475:2401-8. [Crossref] [PubMed]
- DePhillipo NN, Moatshe G, Brady A, et al. Effect of Meniscocapsular and Meniscotibial Lesions in ACL-Deficient and ACL-Reconstructed Knees: A Biomechanical Study. Am J Sports Med 2018;46:2422-31. [Crossref] [PubMed]
- Schindler OS. Surgery for anterior cruciate ligament deficiency: A historical perspective. Knee Surg Sports Traumatol Arthrosc 2012;20:5-47. [Crossref] [PubMed]
- Ellison AE. Anterolateral rotatory instability. In: Funk FJ Jr. editor. Symposium on the Athlete's Knee, Surgical Repair and Reconstruction. Vol American Academy of Orthopaedic Surgeons; 1978:178-93.
- Sydney S, Haynes D, Hungerford D, et al. The altered kinematic effect of an ilitotibial band tenodesis on the anterior cruciate deficient knee. Orthop Res Soc Annu Meet 1987. p. 340.
- Draganich LF, Reider B, Miller PR. An in vitro study of the Muller anterolateral femorotibial ligament tenodesis in the anterior cruciate ligament deficient knee. Am J Sports Med 1989;17:357-62. [Crossref] [PubMed]
- Amis AA, Scammell BE. Biomechanics of intra-articular and extra-articular reconstruction of the anterior cruciate ligament. J Bone Joint Surg Br 1993;75:812-7. [Crossref] [PubMed]
- Devitt BM, Bouguennec N, Barfod KW, et al. Combined anterior cruciate ligament reconstruction and lateral extra-articular tenodesis does not result in an increased rate of osteoarthritis: a systematic review and best evidence synthesis. Knee Surg Sports Traumatol Arthrosc 2017;25:1149-60. [Crossref] [PubMed]
- Hewison CE, Tran MN, Kaniki N, et al. Lateral extra-articular tenodesis reduces rotational laxity when combined with anterior cruciate ligament reconstruction: a systematic review of the literature. Arthroscopy 2015;31:2022-34. [Crossref] [PubMed]
- Zaffagnini S, Marcheggiani Muccioli GM, Grassi A, et al. Over-the-top ACL Reconstruction Plus Extra-articular Lateral Tenodesis With Hamstring Tendon Grafts: Prospective Evaluation With 20-Year Minimum Follow-up. Am J Sports Med 2017;45:3233-42. [Crossref] [PubMed]
- Sonnery-Cottet B, Barbosa NC, Tuteja S, et al. Minimally invasive anterolateral ligament reconstruction in the setting of anterior cruciate ligament injury. Arthrosc Tech 2016;5:e211-5. [Crossref] [PubMed]
- Helito CP, Bonadio MB, Gobbi RG, et al. Combined intra- and extraarticular reconstruction of the anterior cruciate ligament: the reconstruction of the knee anterolateral ligament. Arthrosc Tech 2015;4:e239-44. [Crossref] [PubMed]
- Smith JO, Yasen SK, Lord B, et al. Combined anterolateral ligament and anatomic anterior cruciate ligament reconstruction of the knee. Knee Surg Sports Traumatol Arthrosc 2015;23:3151-6. [Crossref] [PubMed]
- Chahla J, Menge TJ, Mitchell JJ, et al. Anterolateral Ligament Reconstruction Technique: An Anatomic-Based Approach. Arthrosc Tech 2016;5:e453-7. [Crossref] [PubMed]
- Schon JM, Moatshe G, Brady AW, et al. Anatomic Anterolateral Ligament Reconstruction of the Knee Leads to Overconstraint at Any Fixation Angle. Am J Sports Med 2016;44:2546-56. [Crossref] [PubMed]
- Inderhaug E, Stephen JM, Williams A, et al. Biomechanical Comparison of Anterolateral Procedures Combined With Anterior Cruciate Ligament Reconstruction. Am J Sports Med 2017;45:347-54. [Crossref] [PubMed]
- Kato Y, Maeyama A, Lertwanich P, et al. Biomechanical comparison of different graft positions for single-bundle anterior cruciate ligament reconstruction. Knee Surg Sports Traumatol Arthrosc 2013;21:816-23. [Crossref] [PubMed]
- Engebretsen L, Lew WD, Lewis JL, et al. The effect of an iliotibial tenodesis on intraarticular graft forces and knee joint motion. Am J Sports Med 1990;18:169-76. [Crossref] [PubMed]
- Monaco E, Labianca L, Conteduca F, et al. Double bundle or single bundle plus extraarticular tenodesis in ACL reconstruction? Knee Surg Sports Traumatol Arthrosc 2007;15:1168-74. [Crossref] [PubMed]
- Zaffagnini S, Signorelli C, Lopomo N, et al. Anatomic double-bundle and over-the-top single-bundle with additional extra-articular tenodesis: an in vivo quantitative assessment of knee laxity in two different ACL reconstructions. Knee Surg Sports Traumatol Arthrosc 2012;20:153-9. [Crossref] [PubMed]
- Geeslin AG, Moatshe G, Chahla J, et al. Anterolateral knee extra-articular stabilizers: a robotic study comparing anterolateral ligament reconstruction and modified Lemaire lateral extra-articular tenodesis. Am J Sports Med 2018;46:607-16. [Crossref] [PubMed]
- Rezende FC, de Moraes VY, Martimbianco ALC, et al. Does combined intra and extraarticular ACL reconstruction improve function and stability? A meta-analysis. Clin Orthop Relat Res 2015;473:2609-18. [Crossref] [PubMed]
- Song GY, Hong L, Zhang H, et al. Clinical outcomes of combined lateral extra-articular tenodesis and intra-articular anterior cruciate ligament reconstruction in addressing high-grade pivot-shift phenomenon. Arthroscopy 2016;32:898-905. [Crossref] [PubMed]
- Williams A, Ball S, Stephen J, et al. The scientific rationale for lateral tenodesis augmentation of intra-articular ACL reconstruction using a modified 'Lemaire' procedure. Knee Surg Sports Traumatol Arthrosc 2017;25:1339-44. [Crossref] [PubMed]
- Sonnery-Cottet B, Saithna A, Cavalier M, et al. Anterolateral ligament reconstruction is associated with significantly reduced ACL graft rupture rates at a minimum follow-up of 2 years: a prospective comparative study of 502 patients from the SANTI study group. Am J Sports Med 2017;45:1547-57. [Crossref] [PubMed]
- Thaunat M, Clowez G, Saithna A, et al. Reoperation Rates After Combined Anterior Cruciate Ligament and Anterolateral Ligament Reconstruction: A Series of 548 Patients From the SANTI Study Group With a Minimum Follow-up of 2 Years. Am J Sports Med 2017;45:2569-77. [Crossref] [PubMed]
- Sonnery-Cottet B, Saithna A, Blakeney WG, et al. Anterolateral Ligament Reconstruction Protects the Repaired Medial Meniscus: A Comparative Study of 383 Anterior Cruciate Ligament Reconstructions From the SANTI Study Group With a Minimum Follow-up of 2 Years. Am J Sports Med 2018;46:1819-26. [Crossref] [PubMed]
- Pernin J, Verdonk P, Si Selmi TA, et al. Long-term follow-up of 24.5 years after intra-articular anterior cruciate ligament reconstruction with lateral extra-articular augmentation. Am J Sports Med 2010;38:1094-102. [Crossref] [PubMed]
Cite this article as: Tulloch S, Getgood A. Consideration of lateral augmentation in anatomic anterior cruciate ligament reconstruction. Ann Joint 2019;4:15.