Anatomic anterior cruciate ligament reconstruction using the quadriceps tendon
Introduction
Anterior cruciate ligament (ACL) injuries are the most common ligament injuries, with an incidence of 100,000 to 200,000 per year (1). ACL reconstruction surgery has achieved largely satisfactory outcomes in 75% to 95% of patients. For a successful ACL reconstruction, choice of graft, anatomical tunnel positioning, adequate footprint coverage, and prevention of complications and morbidity are important issues to be considered.
Currently, bone-patellar tendon-bone (BPTB) and hamstring tendon (HT) autografts are most commonly used in ACL reconstruction (2,3). ACL reconstruction using the quadriceps tendon (QT) was firstly introduced by Marshall et al. (4) and Blauth (5). Staubli and Jakob (6) reported that the QT is characterized by adequate substance and mechanical properties with minimal donor site morbidity such that it is feasible for use as a graft in ACL reconstruction. A survey across 20 countries conducted in 2014 found that the QT was used in 11% of ACL reconstructions (7). A recent systematic review (3) concluded that the QT is a reliable and reproducible graft that should be considered in ACL reconstruction. The increase of QT use in ACL reconstruction could be predicted given its excellent mechanical characteristics, attributed its larger cross-sectional area compared to the patellar tendon (PT) (8,9). Furthermore, a systematic review found that the donor-site morbidity was minimal with QT (2).
The concept of anatomic ACL reconstruction has been well established and has achieved good clinical outcomes. Previous studies have demonstrated better knee stability and function according to anatomic positioning of the femoral bone tunnels in ACL reconstruction than isometric, vertical positioning of the tunnels (7,10-12). Anatomic ACL reconstruction can be defined as an operation using the anatomic insertion area of both the tibial and femoral origin of the ACL for positioning of the tunnels. A limitation of the traditional transtibial technique is that the femoral tunnel position is constrained greatly by the position and angle of the tibial tunnel. The femoral tunnel can be made in a position superior to the native femoral insertion, which results in a vertical graft orientation that leads to residual rotational laxity (10). This has led to a preference for the trans-portal technique or the outside-in technique, which allow to easily creating the tunnel in an anatomical position. However, both techniques also present some disadvantages. When applying the anteromedial trans-portal technique, deep knee bending is required while creating a femoral tunnel, which results in a difficulty maintaining good arthroscopic view and short femoral tunnel (13). Moreover, there is a risk of cartilage damage to the medial femoral condyle. The outside-in technique also makes an acute femoral tunnel obliquity and an abrupt graft bending angle (GBA), which has been suggested to be a biomechanical factor contributing to poor graft healing or graft failure (14). We have modified the traditional transtibial technique by applying a forward drawer force, a varus force, and an external rotational force to the proximal tibia and rotating the femoral guide, followed by insertion of the femoral guide pin.
The most frequent complications of ACL reconstruction include loss of quadriceps and hamstring strength, extension deficit, and anterior knee pain (15,16), with a higher incidence of these complications observed when using BPTB. The quadrupled HT is widely used due to high load to failure (>4,000 N), low morbidity rates, and small incisions (17). However, some authors have reported tunnel widening relying on tendon-to-bone healing and increased failure of HT grafts compared to BPTB grafts (18,19). Comparative studies with the QT and BPTB graft in ACL reconstruction found similar good results in graft stability (20-22), and favorable functional outcomes with the QT graft (smaller thigh strength deficit and less donor site morbidity) (23,24). Moreover, the QT harvest induces minimal quadriceps inhibition and the residual strength of the extensor mechanism is less impaired by a QT harvest than by harvest of a BPTB graft.
Herein, a single-bundle and modified transtibial anatomical ACL reconstruction using the QT graft will be introduced in detail, and clinical outcomes and usefulness of anatomical ACL reconstruction using the QT graft will be reviewed.
Anatomy
The QT graft presents many anatomical advantages for primary ACL reconstruction. It has a larger cross-sectional area compared to other grafts (25). Harris et al. (26) reported that the QT was 1.8 times thicker than the PT. The QT is average 30 mm wide enough to allow harvesting 10-mm wide grafts. The variability in the cross-sectional area has been reported to be similar in both tendons (27).
Staubli and Jakob (6) have indicated that the QT has a wide attachment to the patella. In anatomical studies, the mean lengths of the QT and PT averaged 85–87 and 51–52 mm, respectively (8). The mean cross-sectional area of the QT graft with 10 mm width was measured as 64.6±8.4 mm2, which is considerably larger than the mean values of the PT, at 36.8±5.7 mm2.
Recently, preoperative magnetic resonance imaging (MRI) has been explored as a suitable tool for predicting intraoperative graft size for ACL reconstruction (28). Comparing preoperative MRI measurements with direct intraoperative measurements, the correlation was very highly positive for the QT, highly positive for the PT, negligible-highly positive for the semitendinosus-only tendon, and negligible-moderately positive for the gracilis-only tendon. The QT has also been shown to be a promising graft option particularly for patients with predicted insufficient hamstring grafts (29). All 54 knees had predicted QT graft diameters of >8 mm.
One study reported a histological comparison of the QT and PT to show that the QT comprised 20% more collagen than the PT, suggesting the superiority of the QT as an ACL graft (30).
Biomechanical properties
Biomechanical and cadaveric studies found that normal ACL has an ultimate load to failure of 1,725–2,160 N, and the tensile strengths of intact QT are averaged as 3,660±830 N, which is 90% higher than those of intact PT (17,31,32). Shani et al. (27) reported that ultimate strain was similar between the QT (10.7% change) and the PT (11.4% change). Young’s modulus of elasticity of the QT was significantly smaller than that of the PT. The mean stiffness of the QT was larger than that of the PT.
Surgical techniques
After anesthesia, a complete physical and arthroscopic examination is performed to evaluate the torn ligament and other intra-articular lesions. Next, all additional procedures are performed before the ACL reconstruction. Following the arthroscopic confirmation of a complete ACL rupture, a QT-patellar bone (QTPB) autograft from the ipsilateral limb is harvested.
Harvest of the QTPB autograft
The QTPB is harvested through 4–6 cm midline incision over the middle of the proximal border of the patella. The graft consists of a proximal patellar bone plug and the central QT. Keeping the knee flexed to 80° facilitates the harvest by maintaining tension on the QT. A 10 mm wide, 20 mm long, 7–8 mm thick trapezoidal bone block is obtained from the proximal patella using a saw and osteotome. Next, a 10 mm wide, 6 to 7 mm thick, and 70 to 80 mm long strip of the QT including the full thickness of the rectus femoris tendon and partial thickness of the vastus intermedius tendon is excised in continuity with the patellar bone block initially using a 10-mm Harvester (ConMed Linvatec, Largo, FL, USA) and then finishing with Metzenbaum scissors. Care is taken not to enter the suprapatellar pouch. If entry occurs, the synovial membrane is repaired with an absorbable suture. The superficial layer of the remaining tendon is closed transversely with absorbable closing sutures. The patellar bone defect is not grafted.
Graft preparation
The QTPB graft is prepared to allow passage through 10-mm-diameter tunnels. The bone plug is trimmed to a bullet shape using a saw and a rongeur. The bone block from proximal patella is perforated transversely with drill, and two absorbable sutures are passed through the transverse holes. The tendinous portion of the graft is secured with No. 5 EthibondTM sutures (Ethicon, Somerville, NJ, USA) using Krackow-type stitches leaving approximately 30 mm intra-articular portion (Figure 1).
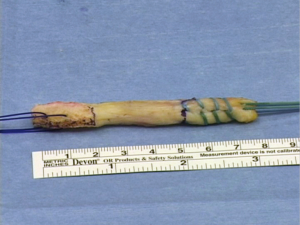
Tunnel placement (a modified trans-tibial technique)
Every effort is made to preserve the remnant tissues of the ruptured ACL as much as possible. However, a remnant may be sacrificed if the preservation is impossible or not useful. The conventional transtibial technique is modified to create the femoral tunnel in the anatomical position (Figure 2). A 3-cm longitudinal skin incision is made at the anteromedial side of the proximal tibia. The entry point of the tibial tunnel is created 4–5 cm distal to the medial tibial plateau, 2–3 cm medial to the tibial tuberosity, just superior to the attachment of the pes anserinus, and just anterior to the medial collateral ligament. Using a tibial drill guide (Acufex, Andover, MA, USA) a guide pin is inserted at an angle of 60° to the tibial plateau, which is aimed at the center of the ACL tibial footprint between the anteromedial and posterolateral bundles. A 10-mm tibial tunnel is made along the guide pin using a cannulated reamer. To create the femoral tunnel, a 7-mm offset femoral guide (Acufex) is directed at the center of the ACL femoral footprint (the lateral bifurcate ridge on the inner wall of the lateral femoral condyle) through the tibial tunnel with the knee flexed to 90° and applying an anterior drawer force to the proximal tibia, a varus force, and an external rotation force to the lower leg while externally rotating the guide (Figure 3). Applying a varus force to the lower leg, with the thigh fixed to the leg holder, provides lateral opening of the knee joint, which enables to aim the femoral guide to the anatomical footprint. Then, a femoral tunnel guide pin is inserted through the guide and a 10-mm-diameter, 20- or 25-mm-long femoral tunnel is drilled through the tibial tunnel using a cannulated reamer. Next, a slot is created for the screw guide pin on the anterior aspect of the femoral tunnel.
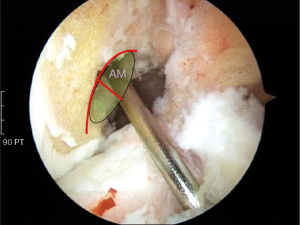
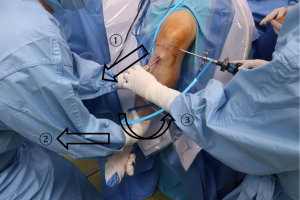
Piasecki et al. (33) has made an effort to modify the trans-tibial technique by making the entry point of the tibial tunnel far medial and proximal to achieve a more oblique trajectory of the ACL graft. However, this modification has resulted in other problems, a shorter tibial tunnel and intra-articular aperture widening to an elliptical shape (34). Previously, we have compared the anteromedial trans-portal technique and the modified trans-tibial technique, and reported the radiological and clinical comparison results (12). The mean values of the angle between the longitudinal axis of the femoral tunnel and the anteroposterior axis of the distal femur on oblique computed tomography (CT) views in the patients treated with our modified trans-tibial technique were significantly smaller than in the patients treated with the anteromedial trans-portal technique. However, there was no significant difference between the two groups in the mean values of the angle between the joint line and the graft (graft obliquity) on coronal and sagittal CT images. The mean femoral tunnel length was significantly greater in the modified trans-tibial technique group compared with the anteromedial trans-portal technique group. Evaluated using the quadrant method, the femoral tunnel position was slightly anterior and inferior in the modified trans-tibial technique group. However, the mean difference in tunnel position between the 2 groups was less than 2 mm and not statistically significant. Regarding the tibial tunnel, the 2 groups showed no significant differences in the ratio of the long-axis length to the short-axis length or the area of the intra-articular aperture, which was checked because of concerns of resultant intra-articular aperture widening due to the modified trans-tibial technique. The tibial tunnel length was slightly shorter in the modified trans-tibial technique; however, the mean length was over 32 mm (minimum 28 mm), which was enough for graft fixation and healing. There were no significant differences in the clinical results (joint stability using manual laxity tests and arthrometry or clinical scores) between the 2 groups.
Graft fixation
Secure graft fixation, graft tension during fixation, and graft fixation level are crucial aspects in ACL reconstruction. The bone block was inserted to the femoral tunnel with the bony part facing forward. Then a metal interference screw (ConMed Linvatec) is used to fix the bone block with the knee flexed. On the tibial side, the tendinous portion of the graft is firstly fixed with a bioabsorbable screw (BioScrew; ConMed Linvatec) in the tibial tunnel without any rotation of the graft and is tightened by tying sutures over a bicortical screw, which is inserted 1–2 cm inferior to the tibial tunnel with the knee extended.
Rehabilitation
Immediately after surgery, full extension was achieved, and full flexion was obtained by 6 weeks. A motion-controlled brace set at 0° to 90° was applied for 4 weeks, then 0° to full flexion for an additional 2 months postoperatively. Partial weight-bearing was permitted for 6 weeks and progressed as tolerated. Full strenuous activity and sports were allowed after 6 months postoperatively, confirming the recovery of quadriceps muscle strength.
Clinical results
The QT autograft has been gaining support from recent studies including systematic reviews, where it has demonstrated clinical outcomes and biomechanical features similar or superior to those achieved with BPTB and HT grafts (2,3,20,35,36).
Stability
In our case series (21), 227 patients (94.6%) demonstrated grade 0 or 1 laxity on the Lachman test, the anterior drawer test, or the pivot-shift test. KT-1000 arthrometric measurement also showed a significant improvement in side-to-side differences on manual maximum test, with a mean of 2.4±1.7 mm at a minimum 2-year follow-up, at which time 11 knees (5%) showed laxity of more than 5 mm. A recent systematic review including 8 studies (1 level II, 7 level III) and 368 patients that underwent primary ACL reconstruction with QT autografts, including 225 patients with BPTB and 150 patients with HT, showed that QT patients showed less knee laxity postoperatively compared with HT patients. However, there were no significant differences in graft failure rates between groups (36).
Functional outcome
In 2004, we reported that ACL reconstruction using a QT autograft resulted in satisfactory clinical outcomes with reduced donor-site morbidity (22). Sixty-seven ACL reconstructions were evaluated at minimum 2 years follow-up. The Lysholm score improved from 71 to 90, postoperatively. In the International Knee Documentation Committee (IKDC) scale, 94% of patients were grade A or B. The peak extension torque of the quadriceps muscle was found to be 82% and 89% of that of the contralateral knee at 1 and 2 years after surgery, respectively. The patellar position showed no significant change after the QT autograft was harvested. Only 6% of patients complained of difficulty in stair-climbing and anterior knee pain, and one patient complained of harvest-site tenderness.
Few studies have reported long-term results. Howe et al. (37) reported a 10-year (mean, 5.5 years) follow-up study of 83 patients with ACL reconstructions using the QT graft. Of these, 92% were satisfied with the results, 93% reported no significant pain, and 92% had no more than a mild dysfunction. Chen et al. (38) evaluated 34 patients with a 4–7-year follow-up, and reported 94% good and excellent results on the Lysholm score. Return to moderate or strenuous sports was possible in 76% of patients. In the IKDC scale, 91% of these patients reported a normal or nearly normal knee.
Several systematic reviews and comparative studies have concluded that the QT autograft achieved clinical (stability) and functional outcomes similar to those reported for BPTB and HT grafts (2,3,36,39,40).
Muscle strength recovery
Although low incidence of anterior knee pain has been consistently reported as an advantage of the QT graft, the effects deriving from the graft harvest on the extensor mechanism have been a relevant concern. Joseph et al. (41) prospectively compared early physical findings of ACL reconstruction using QT, HT, and BPTB grafts. They reported that the QT group achieved knee extension earlier than those reconstructed with BPTB and required less pain medication postoperatively than either the HT or BPTB group. In another study, the QT group had better outcomes in extensor mechanism strength at postoperative 6 months (23).
It is known that knee extensor strength is impaired for more than 1 year after ACL reconstruction, which is especially true when BPTB grafts are used (42-45). The extensor mechanism of the knee is weakened not only following the use of either the BPTB or QT, but also after ACL surgery with HT autografts (46,47). Conversely, knee flexor strength is negatively influenced by the use of HT, whereas QT or BPTB grafts have only a temporary effect (48,49). As against previous finding (50), no evidence for improvement of knee flexor strength over time has been detected in the injured side after HT graft harvesting (51).
In 20 patients undergoing anatomical single-bundle ACL reconstruction using a QT autograft, the average quadriceps strength changed from preoperative 90.5% to 85.1% after 12 months (52). Within 6 months after surgery, quadriceps hypotrophy was observed in all subjects, which nevertheless recovered after postoperative 1 year. No patients complained of donor site pain postoperatively.
Complications and morbidity
Postoperative donor-site morbidity and anterior knee pain following ACL reconstruction result in functional impairment. Injury to the infrapatellar branch of the saphenous nerve in PT harvesting causes paresthesia or numbness on anterior aspect of the knee and is correlated with an inability to kneel. The remaining PT at the donor site exhibited significant clinical, radiological, and histological abnormalities 2 years after harvest (53). Previous studies found lower incidences of sensory loss, anterior knee pain, and donor-site morbidity after QT harvesting when compared with BPTB or HT harvesting (35,53-55). We have previously reported that the prevalence of a patellar fracture was below 2% when using a QT autograft (22).
The tensile strength of the remaining QT and PT after harvesting was measured and compared with that of intact QT and PT in a cadaveric study (32). Removing a 10-mm-wide graft from the distal QT reduced average 34% of tensile strength. The strength of the QT after graft harvesting was higher than that of the intact PT, which suggests that the risk of extensor mechanism rupture may be less in QT than in PT.
Harvesting the HT autograft can cause complications of injury to the surrounding neurovascular structures, tendon amputation during the harvest, and a decrease in knee flexor strength (53). Furthermore, complications including medial thigh and calf hematoma and spasm pain are common after harvesting the HT autografts (56). The advantages of QT autografting include preservation of flexor function, early achievement of knee extensor strength, less analgesics requirement, and a reduced incidence of anterior numbness (41,57,58).
Comparative study: versus BPTB autografts
We compared the clinical outcomes following ACL reconstructions using central QTPB and BPTB autografts (20). Seventy-two patients who underwent unilateral ACL reconstruction using BPTB were selected and matched for age and sex with 72 patients receiving QTPB grafts. More patients [28 (39%)] in the BPTB group reported anterior knee pain than in the QTPB group [6 (8.3%)]. Sixty-eight patients (94%) in the BPTB group and 66 (92%) in the QTPB group graded their knees as normal or nearly normal at final follow-up.
Previous studies have compared the effectiveness of QT autografts with those of BPTB autografts and have shown that the QT autograft produced similar outcomes in anteroposterior stability and functional scores (Lysholm and IKDC subjective scores) (2,20,24,59-61). Moreover, patients who underwent ACL reconstruction with QT autografts experienced less anterior knee pain than those with BPTB autografts (20,54,59,60).
Gorschewsky et al. (24) reported similar stability between QT and BPTB in manual tests and in KT-1000 arthrometric measurements. Lund et al. (54) and Kim et al. (60) also reported similar anterior knee laxity. However, they reported that rotational stability evaluated by pivot shift test was better with QT. Geib at al. reported that the QT autograft showed better results, with less anterior knee pain, less anterior numbness, a higher percentage of a side-to-side difference of less than 3 mm in arthrometric measurements, and less extension loss (59). They suggested that the larger cross-sectional area of the QT reduced tunnel-mismatch and tibial tunnel widening (62). Pigozzi et al. (23). Compared the isokinetic results of patients receiving either the BPTB or the QT graft, and concluded that the thigh muscle strength deficit in the QT group was smaller after 6 months postoperatively.
The results of a systematic review suggest that no significant difference existed in the graft failure rate or in patient reported outcomes (PROs) between primary ACL reconstruction with a QT, BPTB, or HT autografts at a minimum 2-year follow-up (36). PROs of QT patients were generally improved more than those of BPTB or HT patients. The most common disadvantages of BPTB autografts include anterior knee pain and knee extensor weakness. Delayed graft incorporation and reduced knee flexor strength have been reported as the main disadvantages of the HT autograft (63,64). Joseph et al. (41) compared QT grafts with two other grafts for ACL reconstruction. They found that the QT group required fewer analgesics and achieved active extension more rapidly than the BPTB group.
Comparative study: versus HT autografts
We have previously reported a cohort study of 96 patients who underwent ACL reconstruction with either double-bundle HT or single-bundle QT autograft (35). The maximum KT-2000 arthrometer side-to-side difference improved in both HT and QT groups. The modified Lysholm scores, IKDC subjective evaluation scores, and Tegner activity scores also improved in both groups. There were no between-group differences in postoperative anterior knee pain, nor were there differences in thigh extensor strength recovery evaluated with isokinetic test. However, thigh flexor strength recovery was better in the QT group. Anatomic ACL reconstruction with the QT autograft showed similar knee stability and functional outcome compared to the HT autograft.
A recent cohort study of 86 patients reported that ACL reconstruction using QT grafts showed equal or better functional outcomes when compared to ACL reconstruction using HT grafts (65). The number of cases with negative Lachman test after surgery was higher in the QT group than in the HT group (90% vs. 46%). Also, the number of cases with negative pivot-shift test was higher in the QT group than in the HT group (90% vs. 64%). Farber et al. (66) reported that hamstring weakness was the greatest concern among surgeons treating elite soccer players. A randomized controlled trial of 56 soccer players comparing HT and QT grafts showed a significant difference in the hamstring/quadriceps (H/Q) ratio and peak torque values in the extensor muscle strength over time (67). ACL reconstruction with a QT graft showed similar functional outcomes with a better isokinetic H/Q ratio compared to ACL reconstruction with the HT at the 1-year follow-up in soccer players.
In a recent systematic review of 15 clinical trials including 1,910 patients, there was no difference in the graft rupture rate among QT, BPTB and HT in any of the studies (39). Two studies reported that QT resulted in better knee stability than BPTB or HT. In addition, 2 studies found that QT resulted in greater functional outcomes than BPTB or HT.
Radiological results
Twenty-six patients subjected anatomic single-bundle ACL reconstruction with either HT or QTPB autografts underwent postoperative MRI 6 months after surgery (68). The maturity of QTPB was better in comparison with HT. Bone-to-bone healing in the femoral tunnel may have a positive effect on the intra-articular graft healing process. During biological graft healing process, the microenvironment around the graft plays an important role. Bone-bone healing is more secure and faster than tendon-to-bone healing (69).
In an in vivo analysis, signal intensities of the reconstructed QT graft were reported to be highest in the proximal region and lowest in the distal region at 6 months postoperatively (14). An acute GBA was correlated with high signal intensity of the proximal graft. The authors suggested that an acute GBA might negatively affect proximal graft healing after ACL reconstruction using QT grafts. We conducted transmission electron microscopic evaluation of the biopsy specimen from QT grafts. Twenty-eight (76%) of the 37 specimens showed the original bimodal pattern of fibrils composed of small and large-diameter fibrils, which may indicate the mechanical superiority of the QT (21).
Conclusions
The single-bundle and modified transtibial anatomical ACL reconstruction using the QT autograft is supported by the current literature. It is a robust, reliable, and reproducible surgical technique and graft choice that can be considered in primary ACL reconstruction.
Acknowledgments
Funding: None.
Footnote
Provenance and Peer Review: This article was commissioned by the Guest Editor (Takeshi Muneta) for the series “Anatomic Reconstruction of Anterior Cruciate Ligament - Concept, Indication, and Its Efficacy” published in Annals of Joint. The article has undergone external peer review.
Conflict of Interest: Both authors have completed the ICMJE uniform disclosure form (available at http://dx.doi.org/10.21037/aoj.2018.12.10). The series “Anatomic Reconstruction of Anterior Cruciate Ligament - Concept, Indication, and Its Efficacy” was commissioned by the editorial office without any funding or sponsorship. MCL serves as an unpaid editorial board member of Annals of Joint from Aug 2017 to Jul 2019. The authors have no other conflicts of interest to declare.
Ethical Statement: The authors are accountable for all aspects of the work in ensuring that questions related to the accuracy or integrity of any part of the work are appropriately investigated and resolved.
Open Access Statement: This is an Open Access article distributed in accordance with the Creative Commons Attribution-NonCommercial-NoDerivs 4.0 International License (CC BY-NC-ND 4.0), which permits the non-commercial replication and distribution of the article with the strict proviso that no changes or edits are made and the original work is properly cited (including links to both the formal publication through the relevant DOI and the license). See: https://creativecommons.org/licenses/by-nc-nd/4.0/.
References
- Kim HS, Seon JK, Jo AR. Current trends in anterior cruciate ligament reconstruction. Knee Surg Relat Res 2013;25:165-73. [Crossref] [PubMed]
- Mulford JS, Hutchinson SE, Hang JR. Outcomes for primary anterior cruciate reconstruction with the quadriceps autograft: a systematic review. Knee Surg Sports Traumatol Arthrosc 2013;21:1882-8. [Crossref] [PubMed]
- Slone HS, Romine SE, Premkumar A, et al. Quadriceps tendon autograft for anterior cruciate ligament reconstruction: a comprehensive review of current literature and systematic review of clinical results. Arthroscopy 2015;31:541-54. [Crossref] [PubMed]
- Marshall JL, Warren RF, Wickiewicz TL, et al. The anterior cruciate ligament: a technique of repair and reconstruction. Clin Orthop Relat Res 1979;97-106. [PubMed]
- Blauth W. 2-strip substitution-plasty of the anterior cruciate ligament with the quadriceps tendon. Unfallheilkunde 1984;87:45-51. [PubMed]
- Stäubli HU, Jakob RP. Central quadriceps tendon for anterior cruciate ligament reconstruction. Part I: morphometric and biochemical evaluation. Am J Sports Med 1997;25:725-7. [PubMed]
- Middleton KK, Hamilton T, Irrgang JJ, et al. Anatomic anterior cruciate ligament (ACL) reconstruction: a global perspective. Part 1. Knee Surg Sports Traumatol Arthrosc 2014;22:1467-82. [Crossref] [PubMed]
- Stäubli HU, Schatzmann L, Brunner P, et al. Quadriceps tendon and patellar ligament: cryosectional anatomy and structural properties in young adults. Knee Surg Sports Traumatol Arthrosc 1996;4:100-10. [Crossref] [PubMed]
- Lubowitz JH. Editorial commentary: Quadriceps tendon autograft use for anterior cruciate ligament reconstruction predicted to increase. Arthroscopy 2016;32:76-7. [Crossref] [PubMed]
- Lee MC, Seong SC, Lee S, et al. Vertical femoral tunnel placement results in rotational knee laxity after anterior cruciate ligament reconstruction. Arthroscopy 2007;23:771-8. [Crossref] [PubMed]
- Crall TS, Gilmer BB. Anatomic all-inside anterior cruciate ligament reconstruction using quadriceps tendon autograft. Arthrosc Tech 2015;4:e841-5. [Crossref] [PubMed]
- Lee JK, Lee S, Seong SC, et al. Anatomic single-bundle ACL reconstruction is possible with use of the modified transtibial technique: a comparison with the anteromedial transportal technique. J Bone Joint Surg Am 2014;96:664-72. [Crossref] [PubMed]
- Lubowitz JH. Anteromedial portal technique for the anterior cruciate ligament femoral socket: pitfalls and solutions. Arthroscopy 2009;25:95-101. [Crossref] [PubMed]
- Tashiro Y, Gale T, Sundaram V, et al. The graft bending angle can affect early graft healing after anterior cruciate ligament reconstruction: in vivo analysis with 2 years' follow-up. Am J Sports Med 2017;45:1829-36. [Crossref] [PubMed]
- Aglietti P, Buzzi R, D'Andria S, et al. Patellofemoral problems after intraarticular anterior cruciate ligament reconstruction. Clin Orthop Relat Res 1993;195-204. [PubMed]
- Sachs RA, Daniel DM, Stone ML, et al. Patellofemoral problems after anterior cruciate ligament reconstruction. Am J Sports Med 1989;17:760-5. [Crossref] [PubMed]
- West RV, Harner CD. Graft selection in anterior cruciate ligament reconstruction. J Am Acad Orthop Surg 2005;13:197-207. [Crossref] [PubMed]
- Andersson D, Samuelsson K, Karlsson J. Treatment of anterior cruciate ligament injuries with special reference to surgical technique and rehabilitation: an assessment of randomized controlled trials. Arthroscopy 2009;25:653-85. [Crossref] [PubMed]
- Persson A, Fjeldsgaard K, Gjertsen JE, et al. Increased risk of revision with hamstring tendon grafts compared with patellar tendon grafts after anterior cruciate ligament reconstruction: a study of 12,643 patients from the Norwegian Cruciate Ligament Registry, 2004-2012. Am J Sports Med 2014;42:285-91. [Crossref] [PubMed]
- Han HS, Seong SC, Lee S, et al. Anterior cruciate ligament reconstruction: quadriceps versus patellar autograft. Clin Orthop Relat Res 2008;466:198-204. [Crossref] [PubMed]
- Lee S, Seong SC, Jo CH, et al. Anterior cruciate ligament reconstruction with use of autologous quadriceps tendon graft. J Bone Joint Surg Am 2007;89:116-26. [PubMed]
- Lee S, Seong SC, Jo H, et al. Outcome of anterior cruciate ligament reconstruction using quadriceps tendon autograft. Arthroscopy 2004;20:795-802. [Crossref] [PubMed]
- Pigozzi F, Di Salvo V, Parisi A, et al. Isokinetic evaluation of anterior cruciate ligament reconstruction: quadriceps tendon versus patellar tendon. J Sports Med Phys Fitness 2004;44:288-93. [PubMed]
- Gorschewsky O, Klakow A, Putz A, et al. Clinical comparison of the autologous quadriceps tendon (BQT) and the autologous patella tendon (BPTB) for the reconstruction of the anterior cruciate ligament. Knee Surg Sports Traumatol Arthrosc 2007;15:1284-92. [Crossref] [PubMed]
- Stäubli HU, Schatzmann L, Brunner P, et al. Mechanical tensile properties of the quadriceps tendon and patellar ligament in young adults. Am J Sports Med 1999;27:27-34. [Crossref] [PubMed]
- Harris NL, Smith DA, Lamoreaux L, et al. Central quadriceps tendon for anterior cruciate ligament reconstruction. Part I: Morphometric and biomechanical evaluation. Am J Sports Med 1997;25:23-8. [Crossref] [PubMed]
- Shani RH, Umpierez E, Nasert M, et al. Biomechanical comparison of quadriceps and patellar tendon grafts in anterior cruciate ligament reconstruction. Arthroscopy 2016;32:71-5. [Crossref] [PubMed]
- Agarwal S, de Sa D, Peterson DC, et al. Can preoperative magnetic resonance imaging predict intraoperative autograft size for anterior cruciate ligament reconstruction? A systematic review. J Knee Surg 2018; [Epub ahead of print]. [Crossref] [PubMed]
- Ashford WB, Kelly TH, Chapin RW, et al. Predicted quadriceps vs. quadrupled hamstring tendon graft size using 3-dimensional MRI. Knee 2018;25:1100-6. [Crossref] [PubMed]
- Hadjicostas PT, Soucacos PN, Koleganova N, et al. Comparative and morphological analysis of commonly used autografts for anterior cruciate ligament reconstruction with the native ACL: an electron, microscopic and morphologic study. Knee Surg Sports Traumatol Arthrosc 2008;16:1099-107. [Crossref] [PubMed]
- Noyes FR, Butler DL, Grood ES, et al. Biomechanical analysis of human ligament grafts used in knee-ligament repairs and reconstructions. J Bone Joint Surg Am 1984;66:344-52. [Crossref] [PubMed]
- Adams DJ, Mazzocca AD, Fulkerson JP. Residual strength of the quadriceps versus patellar tendon after harvesting a central free tendon graft. Arthroscopy 2006;22:76-9. [Crossref] [PubMed]
- Piasecki DP, Bach BR Jr, Espinoza Orias AA, et al. Anterior cruciate ligament reconstruction: can anatomic femoral placement be achieved with a transtibial technique? Am J Sports Med 2011;39:1306-15. [Crossref] [PubMed]
- Heming JF, Rand J, Steiner ME. Anatomical limitations of transtibial drilling in anterior cruciate ligament reconstruction. Am J Sports Med 2007;35:1708-15. [Crossref] [PubMed]
- Lee JK, Lee S, Lee MC. Outcomes of Anatomic Anterior Cruciate Ligament Reconstruction: Bone-Quadriceps Tendon Graft Versus Double-Bundle Hamstring Tendon Graft. Am J Sports Med 2016;44:2323-9. [Crossref] [PubMed]
- Belk JW, Kraeutler MJ, Marshall HA, et al. Quadriceps tendon autograft for primary anterior cruciate ligament reconstruction: a systematic review of comparative studies with minimum 2-year follow-up. Arthroscopy 2018;34:1699-707. [Crossref] [PubMed]
- Howe JG, Johnson RJ, Kaplan MJ, et al. Anterior cruciate ligament reconstruction using quadriceps patellar tendon graft. Part I. Long-term followup. Am J Sports Med 1991;19:447-57. [Crossref] [PubMed]
- Chen CH, Chuang TY, Wang KC, et al. Arthroscopic anterior cruciate ligament reconstruction with quadriceps tendon autograft: clinical outcome in 4-7 years. Knee Surg Sports Traumatol Arthrosc 2006;14:1077-85. [Crossref] [PubMed]
- Hurley ET, Calvo-Gurry M, Withers D, et al. Quadriceps tendon autograft in anterior cruciate ligament reconstruction: a systematic review. Arthroscopy 2018;34:1690-8. [Crossref] [PubMed]
- Reinhardt KR, Hetsroni I, Marx RG. Graft selection for anterior cruciate ligament reconstruction: a level I systematic review comparing failure rates and functional outcomes. Orthop Clin North Am 2010;41:249-62. [Crossref] [PubMed]
- Joseph M, Fulkerson J, Nissen C, et al. Short-term recovery after anterior cruciate ligament reconstruction: a prospective comparison of three autografts. Orthopedics 2006;29:243-8. [PubMed]
- Kanamoto T, Tanaka Y, Yonetani Y, et al. Anterior knee symptoms after double-bundle ACL reconstruction with hamstring tendon autografts: an ultrasonographic and power Doppler investigation. Knee Surg Sports Traumatol Arthrosc 2015;23:3324-9. [Crossref] [PubMed]
- Lautamies R, Harilainen A, Kettunen J, et al. Isokinetic quadriceps and hamstring muscle strength and knee function 5 years after anterior cruciate ligament reconstruction: comparison between bone-patellar tendon-bone and hamstring tendon autografts. Knee Surg Sports Traumatol Arthrosc 2008;16:1009-16. [Crossref] [PubMed]
- Neeter C, Gustavsson A, Thomee P, et al. Development of a strength test battery for evaluating leg muscle power after anterior cruciate ligament injury and reconstruction. Knee Surg Sports Traumatol Arthrosc 2006;14:571-80. [Crossref] [PubMed]
- Xergia SA, McClelland JA, Kvist J, et al. The influence of graft choice on isokinetic muscle strength 4-24 months after anterior cruciate ligament reconstruction. Knee Surg Sports Traumatol Arthrosc 2011;19:768-80. [Crossref] [PubMed]
- Aglietti P, Giron F, Buzzi R, et al. Anterior cruciate ligament reconstruction: bone-patellar tendon-bone compared with double semitendinosus and gracilis tendon grafts. A prospective, randomized clinical trial. J Bone Joint Surg Am 2004;86-A:2143-55. [Crossref] [PubMed]
- Beard DJ, Anderson JL, Davies S, et al. Hamstrings vs. patella tendon for anterior cruciate ligament reconstruction: a randomised controlled trial. Knee 2001;8:45-50. [Crossref] [PubMed]
- Ageberg E, Roos HP, Silbernagel KG, et al. Knee extension and flexion muscle power after anterior cruciate ligament reconstruction with patellar tendon graft or hamstring tendons graft: a cross-sectional comparison 3 years post surgery. Knee Surg Sports Traumatol Arthrosc 2009;17:162-9. [Crossref] [PubMed]
- Heijne A, Werner S. A 2-year follow-up of rehabilitation after ACL reconstruction using patellar tendon or hamstring tendon grafts: a prospective randomised outcome study. Knee Surg Sports Traumatol Arthrosc 2010;18:805-13. [Crossref] [PubMed]
- Ardern CL, Webster KE, Taylor NF, et al. Hamstring strength recovery after hamstring tendon harvest for anterior cruciate ligament reconstruction: a comparison between graft types. Arthroscopy 2010;26:462-9. [Crossref] [PubMed]
- Fischer F, Fink C, Herbst E, et al. Higher hamstring-to-quadriceps isokinetic strength ratio during the first post-operative months in patients with quadriceps tendon compared to hamstring tendon graft following ACL reconstruction. Knee Surg Sports Traumatol Arthrosc 2018;26:418-25. [Crossref] [PubMed]
- Iriuchishima T, Ryu K, Okano T, et al. The evaluation of muscle recovery after anatomical single-bundle ACL reconstruction using a quadriceps autograft. Knee Surg Sports Traumatol Arthrosc 2017;25:1449-53. [Crossref] [PubMed]
- Kartus J, Movin T, Karlsson J. Donor-site morbidity and anterior knee problems after anterior cruciate ligament reconstruction using autografts. Arthroscopy 2001;17:971-80. [Crossref] [PubMed]
- Lund B, Nielsen T, Fauno P, et al. Is quadriceps tendon a better graft choice than patellar tendon? a prospective randomized study. Arthroscopy 2014;30:593-8. [Crossref] [PubMed]
- Sofu H, Sahin V, Gursu S, et al. Use of quadriceps tendon versus hamstring tendon autograft for arthroscopic anterior cruciate ligament reconstruction: a comparative analysis of clinical results. Eklem Hastalik Cerrahisi 2013;24:139-43. [Crossref] [PubMed]
- Carofino B, Fulkerson J. Medial hamstring tendon regeneration following harvest for anterior cruciate ligament reconstruction: fact, myth, and clinical implication. Arthroscopy 2005;21:1257-65. [Crossref] [PubMed]
- Slullitel D, Blasco A, Periotti G. Full-thickness quadriceps tendon: An easy cruciate reconstruction graft. Arthroscopy 2001;17:781-3. [Crossref] [PubMed]
- Guimarães MV, Junior LH, Terra DL. Reconstruction of the anterior cruciate ligament with the central third of the quadriceps muscle tendon: analysis of 10-year results. Rev Bras Ortop 2015;44:306-12. [PubMed]
- Geib TM, Shelton WR, Phelps RA, et al. Anterior cruciate ligament reconstruction using quadriceps tendon autograft: intermediate-term outcome. Arthroscopy 2009;25:1408-14. [Crossref] [PubMed]
- Kim SJ, Kumar P, Oh KS. Anterior cruciate ligament reconstruction: autogenous quadriceps tendon-bone compared with bone-patellar tendon-bone grafts at 2-year follow-up. Arthroscopy 2009;25:137-44. [Crossref] [PubMed]
- Xerogeanes JW, Mitchell PM, Karasev PA, et al. Anatomic and morphological evaluation of the quadriceps tendon using 3-dimensional magnetic resonance imaging reconstruction: applications for anterior cruciate ligament autograft choice and procurement. Am J Sports Med 2013;41:2392-9. [Crossref] [PubMed]
- Shino K, Oakes BW, Horibe S, et al. Collagen fibril populations in human anterior cruciate ligament allografts. Electron microscopic analysis. Am J Sports Med 1995;23:203-8; discussion 209. [Crossref] [PubMed]
- Fineberg MS, Zarins B, Sherman OH. Practical considerations in anterior cruciate ligament replacement surgery. Arthroscopy 2000;16:715-24. [Crossref] [PubMed]
- Aune AK, Holm I, Risberg MA, et al. Four-strand hamstring tendon autograft compared with patellar tendon-bone autograft for anterior cruciate ligament reconstruction. A randomized study with two-year follow-up. Am J Sports Med 2001;29:722-8. [Crossref] [PubMed]
- Cavaignac E, Coulin B, Tscholl P, et al. Is quadriceps tendon autograft a better choice than hamstring autograft for anterior cruciate ligament reconstruction? A comparative study with a mean follow-up of 3.6 years. Am J Sports Med 2017;45:1326-32. [Crossref] [PubMed]
- Farber J, Harris JD, Kolstad K, et al. Treatment of anterior cruciate ligament injuries by major league soccer team physicians. Orthop J Sports Med 2014;2:2325967114559892 [Crossref] [PubMed]
- Martin-Alguacil JL, Arroyo-Morales M, Martin-Gomez JL, et al. Strength recovery after anterior cruciate ligament reconstruction with quadriceps tendon versus hamstring tendon autografts in soccer players: A randomized controlled trial. Knee 2018;25:704-14. [Crossref] [PubMed]
- Ma Y, Murawski CD, Rahnemai-Azar AA, et al. Graft maturity of the reconstructed anterior cruciate ligament 6 months postoperatively: a magnetic resonance imaging evaluation of quadriceps tendon with bone block and hamstring tendon autografts. Knee Surg Sports Traumatol Arthrosc 2015;23:661-8. [Crossref] [PubMed]
- Papageorgiou CD, Ma CB, Abramowitch SD, et al. A multidisciplinary study of the healing of an intraarticular anterior cruciate ligament graft in a goat model. Am J Sports Med 2001;29:620-6. [Crossref] [PubMed]
Cite this article as: Han HS, Lee MC. Anatomic anterior cruciate ligament reconstruction using the quadriceps tendon. Ann Joint 2019;4:2.