Imaging evaluation of the multiligament injured knee
Imaging anatomy
The basis for high-quality imaging is the knowledge of the anatomy of the structures in focus. This is even more true when dealing with a complex anatomy such as the knee. The focus of this section of the article will be to review the anatomy and imaging of the peripheral ligament structures of the knee. To illustrate such an anatomy, we use high resolution turbo spin echo MRI sequences, which allow greater anatomical detail than the conventional sequences used in clinical practice.
Posterolateral corner
The structures of the PLC provide an important biomechanical role as static and dynamic stabilizers against anterior and posterior translation, varus stress and external rotation. The three most important static stabilizers of the PLC are the fibular or lateral collateral ligament (LCL), the popliteus tendon (PLT) and the popliteofibular ligament (PFL) (Figure 1). Other important static stabilizers are the fabellofibular ligament (FFL), the arcuate ligament and the posterolateral joint capsule. The PLT and muscular complex (PTMC) and the biceps femoris are the major dynamic stabilizers of the PLC. The LCL origin is slightly proximal and posterior to the lateral condyle and courses distally attaching on the superior facet of the fibular head. The PLT origins from the popliteal sulcus, anterior from the LCL origin and courses in an oblique way posterodistally to its distal insertion on the posteromedial tibia. The PFL (Figure 1) originates from the PLT at the musculotendinous junction and inserts onto the posteromedial aspect of the fibular head. The biceps femoris consists of a long head and a short head, each inserting on the fibular head. The short head of the biceps femoris has an anterior arm attaching onto Gerdy’s tubercle (1,2).
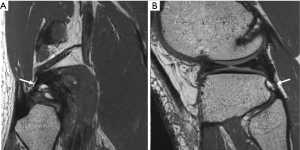
Anterolateral corner
The ALC plays an important role in rotatory knee stability in addition to the ACL. The anterolateral corner main structures contain the superficial and deep portion of the iliotibial band (ITB), including the distal femoral ITB Kaplan fibers (Figure 2), and the anterolateral capsule along with the anterolateral ligament (ALL) (Figure 3), a distinct ligamentous structure within the anterolateral capsule. Although controversy remains as to the role and function of the individual components of the ALC, most authors agree that they work synergistically and all contribute to anterolateral knee stability.
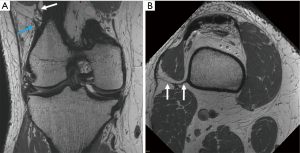
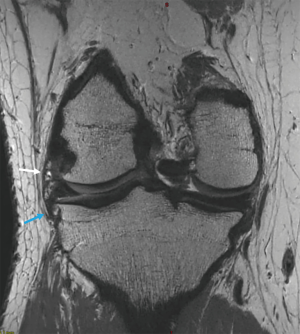
The distal insertion of the ITB is located on the anterolateral tibia, Gerdy’s tubercle. The Kaplan fibers (Figure 2) connect the superficial ITB with the distal femoral metaphysis and condyle, and traverse in close proximity to the superior genicular artery. The ALL (Figure 3) origin is at the lateral femoral epicondyle, often indistinguishable from the LCL origin, and courses anteroinferiorly, bifurcating just above the inferior lateral genicular artery. The ALL inserts onto the lateral meniscus and, mainly, onto the anterolateral tibia, posterior to Gerdy’s tubercle (3-7).
Posteromedial corner (PMC)
The PMC extends posterior from the superficial medial collateral ligament (MCL) to the medial border of the gastrocnemius medial head. The PMC serves as an important support against valgus stress, external and internal rotation, anterior and posterior translation of the knee. The main dynamic stabilizer of the PMC is the semimembranosus tendon (SMT). The most important static stabilizer is the posterior oblique ligament (POL) (Figure 4), also known as ligament of Winslow. Other important structures of the PMC are the oblique popliteal ligament (OPL), posteromedial capsule and the posterior horn of the medial meniscus. The SMT has five major aponeurotic expansions: the direct or principal arm, the capsular arm, the extension to the OPL, the anterior arm and the inferior arm. The direct arm is the most important expansion and inserts below the joint line at the tuberculum tendinis, posteromedial on the medial tibial condyle. The origin of the POL (Figure 4) is located just posterior of the MCL and spreads out posteriorly in an oblique way into three arms. The central arm is the thickest and most important arm, inserting onto the posteromedial aspect of the medial meniscus, subjacent posteromedial tibia and merging with the posteromedial joint capsule. The OPL is different from other structures as it is a supporting component of the PMC and the PLC. The origin of the OPL is on the capsular arm of the POL and the lateral arm of the semimembranosus. It fans out laterally and inserts onto the fabella, posterolateral joint capsule and the plantaris muscle (1,8,9).
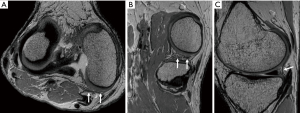
Anteromedial corner (AMC)
The MCL, with its superficial and deep layer (Figure 5), lies between the PMC and AMC. The origin of the superficial MCL is posterior and proximal to the medial epicondyle. It fans out distally and inserts onto the SMT and the posteromedial aspect of the tibia. The deep MCL consists of a meniscofemoral and a shorter meniscotibial component (Figure 5).
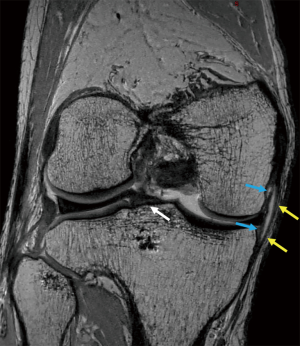
The AMC stretches from the medial border of the patellar tendon to the anterior border of the superficial MCL. The most important stabilizer of the AMC is the medial patellofemoral ligament (MPFL). The MPFL is the primary stabilizer to lateral displacement of the patella. The femoral insertion of the MPFL is located anterior and distal to the adductor tubercle, close to the origin of the superficial MCL. The MPFL courses anterior and fans out inserting onto the medial border of the patella and the deep vastus medialis (9).
Imaging modalities and clinical cases
Imaging modalities
Conventional radiograph (CR) is the first line technique to evaluate acute knee injury. CR is mainly used to screen for fractures and indirect signs of ligamentous injury. A stress CR can demonstrate laxity or instability of the knee (Figure 6). Sonography has limited use in the evaluation of suspected multiligamentous knee injury and should only be used for low impact trauma or specific clinical questions, such as assessment of vascular injuries with color Doppler or peripheral nerve injuries with high frequency probes. Computed tomography (CT) scan can be used to evaluate complex fractures or dislocations (Figure 7), asses vasculature and as problem-solver for doubtful CR. CT arthrography can be useful to evaluate patients with contraindications for MR, but, besides being invasive, is of inferior sensitivity and specificity to evaluate ligamentous injury of the knee (2). MR with its exquisite contrast resolution, is the first choice imaging technique for evaluating the multiligamentous injured knee and for determining the pattern of injury. However, in the acute stage, MR can be difficult to interpret and misleading because of edema and hemorrhage in ligamentous structures. The MR should be performed in the subacute phase to allow edema and hemorrhage to reduce or resolve and thus make ligamentous injury more obvious. In addition to ligament injuries, MR is excellent for assessing concomitant intra-articular lesions and local complications (10).
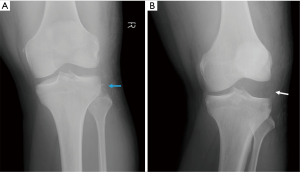
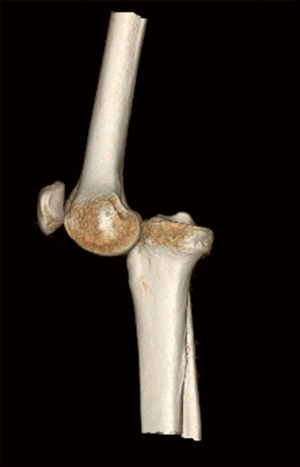
Current knee MR protocols at 1.5 or 3T typically consist of 2D proton density, intermediate- or T2-weighted turbo-spin-echo (TSE) sequences with and without fat-suppression (fs) repeated in multiple planes. Although these sequences provide excellent tissue contrast and high in-plane resolution, they have thick slices resulting in partial volume averaging. Isotropic 3D-TSE sequences are clinically available. These sequences provide thin, continuous slices through the joint, thereby reducing partial volume effects, and allow to create multiplanar reconstructions (10).
Clinical cases
Schenck et al. proposed a combination of clinical and anatomical patterns to categorize KD which has been adopted by the literature and modified (11-13). The classification consists of 5 categories ranging from unicruciate injury with associated ligament tear to KD with periarticular fracture. The system focuses onto the ACL, PCL, MCL, LCL, PLC and periarticular fractures. Recent literature has described the importance of the diagnosis and management of associated ALL and ALC injuries in ACL surgery (14,15) and thus it is our opinion that the ALC should be considered in this modified classification. AMC is of low interest in the femorotibial KD and will not be discussed. In the following, we will illustrate the different classifications with clinical cases.
KD I is defined as KD with an incomplete or complete tear of one of the cruciate ligaments and associated with injury to the peripheral corners. KD I is a frequent sports injury in which the ACL is the most common injured cruciate ligament (Figure 8).
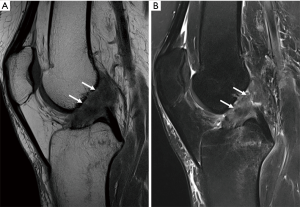
KD II is a rare injury, involving lesions of both the ACL and PCL (Figure 9), but without involvement of the peripheral corners.
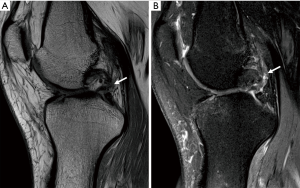
KD III injuries are defined as tears of both cruciate ligaments and one of the collateral ligaments, either medial (KD III M) or lateral (KD III L) (Figure 10), while KD IV injuries are more severe, involving both lateral and medial collateral ligaments (Figure 11). Although classification includes only medial and lateral collateral ligaments, imaging can assess concomitant injuries of posteromedial, posterolateral and anterolateral structures. Medial and lateral collateral ligament injuries prevalence are similar in KD (16), but lateral injuries more frequently involve extra-articular structures.
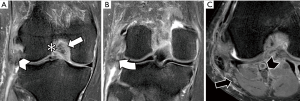
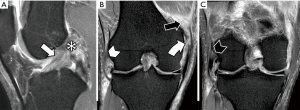
Peripheral ligaments and tendon injuries are depicted as either fiber thickening and adjacent edema (strain), or structural discontinuities (either partial and full thickness tears). Muscular structures may also present injuries at the muscle belly and muscle-tendon junction, either as muscle edema or as tears. Medial and lateral collateral ligaments are depicted in the coronal plane, although axial images are valuable to confirm and assess the extent of injury. Due to popliteus muscle oblique orientation, its tendon injuries are more easily observed in axial and coronal planes, whereas sagittal and axial planes are better to depict muscle belly injuries. PFL and ALL are smaller structures and their evaluation requires great attention. ALL is best assessed in coronal images. Recent studies consider the ALL abnormal when either one of the following are present: discontinuity of fibers, proximal or distal detachment and local edema with fiber irregularity (4,17-22).
KD V is defined as a multiligamentous injury with periarticular fracture. Periarticular fractures are often depicted on CR and include tibia plateau fracture (Figure 12), avulsion fracture of the tibial eminences, Segond fracture or bony avulsion of the ALL (Figure 13), reversed Segond and arcuate sign or avulsion fracture of the fibular head (Figure 14) or a combination (Figure 15). When the bony lesions are small, they are sometimes hard to depict on MR, but the ligamentous injury is usually obvious. On the other hand, bony avulsions with large fragments may change surgical approach, since a fragment reattachment can be performed instead of a ligament reconstruction.
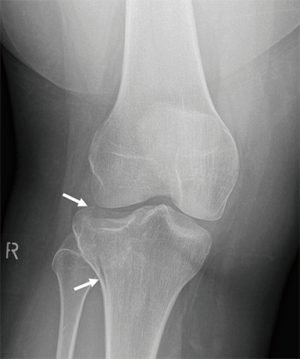
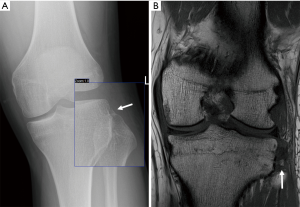
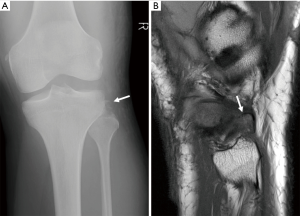
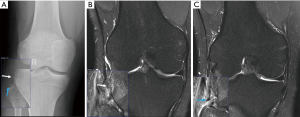
Complications and concomitant lesions
Along with severe central and peripheral ligament injuries, knee dislocations (KDs) often present concomitant injuries with variable severity and morbidity. Of the several lesions associated with multiligamentous knee injuries, it is worth highlighting meniscal and cartilage lesions (Figure 16), due to their high frequency, and neural and vascular lesions (Figure 17), due to potential for patient morbidity.
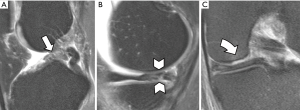
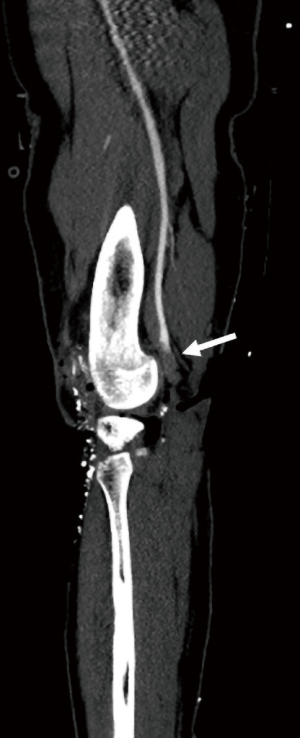
It is worth mentioning that in obese patients KD can occur in lower energy traumas, such as falls from height or traumas in daily activities. These injuries are termed ultra-low velocity dislocations and have some different characteristics from typical high-grade KD, that will be discussed in this chapter.
Meniscus and cartilage injuries
Meniscal injuries are present in 37–57% of dislocated knee injuries (23-25). Predilection for medial or lateral meniscus is variable in the literature, with studies demonstrating equal incidence (24,25), lateral predominance (26), and medial predominance (23). Moatshe et al. compared high energy and low energy KDs and detected a nonsignificant greater odds of meniscal tears in high energy trauma (25).
Articular cartilage injuries are also common, occurring from 28% to 57% of multiligamentous knee injuries (23-25) (Figure 16). Moatshe et al. also described that cartilage injuries were more common at femoral condyles (12.5%) and that patients with meniscal tears and acute ligament tears had greater odds of a cartilage injury (25).
Krych et al. identified that lateral side ligament injuries are more commonly associated with meniscal and cartilage injuries than medial side injuries (24). They attributed that to a higher rate of concomitant lateral meniscus injuries.
MRI is the method of choice to access cartilage and meniscal tears. Sensitivity and specificity of conventional 2D FSE sequences for cartilage lesions is 59–94% and 70–99%, respectively (27-29), and for meniscal tears 72–95% and 85–94%, respectively (30).
Nerve injuries
The common peroneal nerve is the most frequently injured nerve in multiligament knee injuries, occurring in 19–25% of patients (24,31,32). The majority of patients present motor symptoms, isolated or combined with sensory symptoms (25). Tibial nerve injuries, although less common, may also occur, presenting with motor symptoms in 7% of cases (32). The peroneal nerve has a fixed position and goes around the neck of the fibula, which may be responsible for its vulnerability in knee traumas. Peroneal nerve injuries are more common when lateral structures are torn, typically caused by a varus force that stretches the nerve, causing damage from mild elongation to complete transection (33). Peroneal nerve injury is one of the peripheral nerve injuries with worst prognosis, especially high grade injuries which are not eligible to surgical repair (34).
The peroneal nerve can be clearly identified in the posterolateral aspect of the knee at MRI examinations. Becker et al. demonstrated that 70% of peroneal nerve injuries had appropriate MRI findings (32) (Figure 10C). Several imaging signs of peroneal nerve injury have been described in the literature from surrounding edema and mild contusions to encasing hematoma, disruption of fibers and undefined nerve fibers. Potter et al. described that all patients with clinical nerve symptoms presented MRI abnormalities and all patients with severe imaging findings (encasing hematoma, contusion, partial disruption of fibers) presented some degree of symptoms. However, some patients with mild MRI findings (such as surrounding edema or apparent hemorrhage) had no symptoms (23). In addition, Peltola et al. demonstrated a good correlation between normal imaging and absence of clinical signs of peroneal nerve injury (26).
Sonography (US) is also a valuable method for assessing nerve injuries, especially when high-frequency probes are available. Besides excellent resolution and capability of a multiplanar approach, US is not limited to the knee segment, allowing an extensive evaluation nerve path and assessment of muscle denervation. Pathologic US findings include caliber changes, discontinuity, lost fascicular pattern and peripheral hematomas (35).
Patients with fibular head fractures present peroneal neuropraxia or complete motor deficit in near half of cases (32,36).
Werner et al. demonstrated a higher incidence of peroneal nerve injuries in ultra-low velocity KDs compared to all multiligament injured knees (37).
Vascular
Vascular injuries are serious complications of knee trauma and occur in 3.3–18% of patients with multiligament knee injuries and KDs. Those injuries were significantly associated with lateral side ligament injuries, posterior dislocations and peroneal nerve injuries (16,24,31,38-40). In a systematic review by Medina et al., the most common injured vessel was the popliteal artery (76% of cases) (Figure 17), which can be justified by its anatomy, and 80% of vascular injuries demanded repair (31). Of all patients with vascular injury, 12% underwent amputation, the majority due to vascular repair failure (31). The popliteal artery is tethered proximal and distal to the knee joint at the Hunter chanel and at the soleal arch, which are thought to be responsible for the high risk of injury in KDs (32).
Posterior KD results in popliteal artery dissection or transection, while anterior KDs cause traction or stretching trauma, resulting in intimal tears (31,41).
Monitoring of ankle brachial pressure index (ABPI) and distal pulses is the first step for vascular injuries diagnosis. If no abnormality is detected, patients may undergo Doppler sonography or CT angiogram prior to ligament reconstructions. If either distal pulses or ABPI abnormalities are present, conventional angiogram is recommended. Furthermore, whenever there is clear evidence of a nonperfused limb, such as absent distal pulses, immediate intervention is required
As with peroneal nerve injuries, there is a higher incidence of vascular injuries in ultra-low velocity KDs compared to all multiligament injured knees (37).
In the study by Becker et al., compartmental injuries occurred in 16% of patients (32), nearly half of them not associated with revascularization procedure and all of them requiring fasciectomy. Capsular tears may lead to extravasation of arthroscopic fluid to myofascial planes, a potential cause of compartmental syndrome that can be avoided by delaying surgery 10 to 14 days for capsular sealing (42).
Other injuries
Other common concomitant injuries include extensor mechanism tears (8.6%), such as patellar dislocation, and lower extremity fractures (16–58%), more frequently affecting the tibia (25,32). Anterolateral tibia (Segond), tibial spine and fibular head fractures are common examples of avulsion injuries, while tibial plateau fractures represent more serious bone injuries (42).
According to Becker et al. (32), pelvic and spinal fractures occur less frequently in association with multiligament knee injury (12% and 23% of cases in a level I trauma center, respectively).
Non-musculoskeletal concomitant injuries, such as severe head trauma, pulmonary embolism, pneumothorax or hemothorax, intra-abdominal injuries and even death, occur in 10–14% of cases (32), mainly because KDs and multiligament injuries typically occur in high-energy traumas.
Postoperative complications
Postoperative imaging of the multiligament injured knee is challenging, both in terms of imaging acquisition and imaging interpretation. Metallic fragments along instrumentation tracts or material used for graft fixation or ligament reconstruction may cause magnetic susceptibility artifacts, particularly in higher magnetic field strengths, such as 3 tesla MRI scanners. Therefore, the use of metallic artifact reduction sequences, e.g., slice-encoding for metal artifact correction (SEMAC), may be needed (43).
Literature is plentiful regarding postoperative anterior cruciate ligament (ACL) imaging studies, with several studies describing the most common complications such as graft tear, graft impingement and arthrofibrosis (44-49). Literature is restricted regarding posterior cruciate ligament (PCL) reconstruction imaging evaluation and even more scarce when considering medial collateral ligament, posterolateral corner and anterolateral complex repairs (50-53). As a rule, graft presents a higher signal on T2 weighted images, with progressive signal reduction in the first year after surgery. Graft tear is identified as a fiber discontinuity if interposed fluid. Bone marrow edema may be seen near the tunnels, lasting up to 12 months.
Local infection is a described complication related to multiligament knee injury and, although joint aspiration is vital for diagnosis and treatment planning, MRI can suggest and validate the diagnosis. Also, imaging is important to evaluate the severity and extent of the process. MRI findings include joint effusion, synovitis, bone edema, erosions, osteomyelitis, and soft tissue abscesses (48).
Hardware complications, such as fracture and migration are also described (48,51), and may cause graft laxity and intra-articular loose body. ACL graft fixation by endobutton have increased risk of graft cystic degeneration and tunnel widening due to the ‘‘windshield wiper’’ effect (50).
Werner et al. found that complications were more frequent in ultralow velocity multiligament knee injuries (73% vs. 21%), with higher rates of reoperation, infections and joint stiffness (37).
Conclusions
Imaging methods play a key role in complementing clinical assessment of patients with dislocations and multiligament knee injuries. Magnetic resonance imaging (MRI) is the method of choice for its excellent ability to diagnose knee ligament lesions, from the simplest to the most complex, as well as the ability to access intra- and extra-articular structures, including arteries and nerves.
Acknowledgments
Funding: None.
Footnote
Provenance and Peer Review: This article was commissioned by the editorial office, Annals of Joint for the series “The Multiligament Injured Knee”. The article has undergone external peer review.
Conflicts of Interest: All authors have completed the ICMJE uniform disclosure form (available at http://dx.doi.org/10.21037/aoj.2018.09.03). The series “The Multiligament Injured Knee” was commissioned by the editorial office without any funding or sponsorship. CPH served as the unpaid Guest Editor of the series. The authors have no other conflicts of interest to declare.
Ethical Statement: The authors are accountable for all aspects of the work in ensuring that questions related to the accuracy or integrity of any part of the work are appropriately investigated and resolved.
Open Access Statement: This is an Open Access article distributed in accordance with the Creative Commons Attribution-NonCommercial-NoDerivs 4.0 International License (CC BY-NC-ND 4.0), which permits the non-commercial replication and distribution of the article with the strict proviso that no changes or edits are made and the original work is properly cited (including links to both the formal publication through the relevant DOI and the license). See: https://creativecommons.org/licenses/by-nc-nd/4.0/.
References
- Hansford BG, Yablon CM. Multiligamentous Injury of the Knee: MRI Diagnosis and Injury Patterns. Semin Musculoskelet Radiol 2017;21:63-74. [Crossref] [PubMed]
- Naraghi AM, White LM. Imaging of athletic injuries of knee ligaments and menisci: sports imaging series. Radiology 2016;281:23-40. [Crossref] [PubMed]
- Getgood A, Brown C, Lording T, et al. The anterolateral complex of the knee: results from the International ALC Consensus Group Meeting. Knee Surg Sports Traumatol Arthrosc 2018; [Epub ahead of print]. [Crossref] [PubMed]
- Van Dyck P, Clockaerts S, Vanhoenacker FM, et al. Anterolateral ligament abnormalities in patients with acute anterior cruciate ligament rupture are associated with lateral meniscal and osseous injuries. Eur Radiol 2016;26:3383-91. [Crossref] [PubMed]
- Claes S, Vereecke E, Maes M, et al. Anatomy of the anterolateral ligament of the knee. J Anat 2013;223:321-8. [Crossref] [PubMed]
- Godin JA, Chahla J, Moatshe G, et al. A comprehensive reanalysis of the distal iliotibial band: quantitative anatomy, radiographic markers, and biomechanical properties. Am J Sports Med 2017;45:2595-603. [Crossref] [PubMed]
- Van Dyck P, De Smet E, Lambrecht V, et al. The anterolateral ligament of the knee: what the radiologist needs to know. Semin Musculoskelet Radiol 2016;20:26-32. [Crossref] [PubMed]
- Lundquist RB, Matcuk GR, Schein AJ, et al. Posteromedial Corner of the Knee: The Neglected Corner. Radiographics 2015;35:1123-37. [Crossref] [PubMed]
- LaPrade MD, Kennedy MI, Wijdicks CA, et al. Anatomy and Biomechanics of the Medial Side of the Knee and Their Surgical Implications. Sports Med Arthrosc 2015;23:63-70. [Crossref] [PubMed]
- Van Dyck P. Pitfalls in MR imaging of acute anterior cruciate ligament injuries. Knee Surg Sports Traumatol Arthrosc 2017; [Epub ahead of print]. [Crossref] [PubMed]
- Schenck R Jr. Classification of knee dislocations. Oper Tech Sports Med 2003;11:193-8. [Crossref]
- Merritt AL, Wahl C. Initial assessment of the acute and chronic multiple-ligament injured (dislocated) knee. Sports Med Arthrosc Rev 2011;19:93-103. [Crossref] [PubMed]
- Wascher DC, Dvirnak PC, DeCoster TA. Knee dislocation: initial assessment and implications for treatment. J Orthop Trauma 1997;11:525-9. [Crossref] [PubMed]
- Sonnery-Cottet B, Daggett M, Helito CP, et al. Combined Anterior Cruciate Ligament and Anterolateral Ligament Reconstruction. Arthrosc Tech 2016;5:e1253-e1259. [Crossref] [PubMed]
- Helito CP, Saithna A, Bonadio MB, et al. Anterolateral Ligament Reconstruction: A Possible Option in the Therapeutic Arsenal for Persistent Rotatory Instability After ACL Reconstruction. Orthop J Sports Med 2018;6:2325967117751348 [Crossref] [PubMed]
- Bui KL, Ilaslan H, Parker RD, et al. Knee dislocations: A magnetic resonance imaging study correlated with clinical and operative findings. Skeletal Radiol 2008;37:653-61. [Crossref] [PubMed]
- Hartigan DE, Carroll KW, Kosarek FJ, et al. Visibility of Anterolateral Ligament Tears in Anterior Cruciate Ligament-Deficient Knees With Standard 1.5-Tesla Magnetic Resonance Imaging. Arthroscopy 2016;32:2061-5. [Crossref] [PubMed]
- Helito CP, Helito PVP, Leão RV, et al. Anterolateral ligament abnormalities are associated with peripheral ligament and osseous injuries in acute ruptures of the anterior cruciate ligament. Knee Surg Sports Traumatol Arthrosc 2017;25:1140-8. [Crossref] [PubMed]
- Helito CP, Helito PVP, Costa HP, et al. Assessment of the Anterolateral Ligament of the Knee by Magnetic Resonance Imaging in Acute Injuries of the Anterior Cruciate Ligament. Arthroscopy 2017;33:140-6. [Crossref] [PubMed]
- Kosy JD, Schranz PJ, Patel A, et al. The magnetic resonance imaging appearance of the anterolateral ligament of the knee in association with anterior cruciate rupture. Skeletal Radiol 2017;46:1193-200. [Crossref] [PubMed]
- Song GY, Zhang H, Wu G, et al. Patients with high-grade pivot-shift phenomenon are associated with higher prevalence of anterolateral ligament injury after acute anterior cruciate ligament injuries. Knee Surg Sports Traumatol Arthrosc 2017;25:1111-6. [Crossref] [PubMed]
- Song GY, Zhang H, Wang QQ, et al. Bone Contusions After Acute Noncontact Anterior Cruciate Ligament Injury Are Associated With Knee Joint Laxity, Concomitant Meniscal Lesions, and Anterolateral Ligament Abnormality. Arthroscopy 2016;32:2331-41. [Crossref] [PubMed]
- Potter HG, Weinstein M, Allen AA, et al. Magnetic resonance imaging of the multiple-ligament injured knee. J Orthop Trauma 2002;16:330-9. [Crossref] [PubMed]
- Krych AJ, Sousa PL, King AH, et al. Meniscal tears and articular cartilage damage in the dislocated knee. Knee Surg Sports Traumatol Arthrosc 2015;23:3019-25. [Crossref] [PubMed]
- Moatshe G, Dornan GJ, Løken S, et al. Demographics and injuries associated with knee dislocation: A prospective review of 303 patients. Orthop J Sports Med 2017;5:2325967117706521 [Crossref] [PubMed]
- Peltola EK, Lindahl J, Hietaranta H, et al. Knee dislocation in overweight patients. AJR Am J Roentgenol 2009;192:101-6. [Crossref] [PubMed]
- Kijowski R, Blankenbaker DG, Davis KW, et al. Comparison of 1.5-and 3.0-T MR Imaging for Evaluating the Articular Cartilage of the Knee Joint 1 From the Departments of Radiology. Radiology 2009;250:839-48. [Crossref] [PubMed]
- Wong S, Steinbach L, Zhao J, et al. Comparative study of imaging at 3.0 T versus 1.5 T of the knee. Skeletal Radiol 2009;38:761-9. [Crossref] [PubMed]
- Kijowski R. Clinical cartilage imaging of the knee and hip joints. AJR Am J Roentgenol 2010;195:618-28. [Crossref] [PubMed]
- De Smet AA, Tuite MJ. Use of the “Two-Slice-Touch” Rule for the MRI Diagnosis of Meniscal Tears. AJR Am J Roentgenol 2006;187:911-4. [Crossref] [PubMed]
- Medina O, Arom GA, Yeranosian MG, et al. Vascular and nerve injury after knee dislocation: A systematic review. Clin Orthop Relat Res 2014;472:2621-9. [Crossref] [PubMed]
- Becker EH, Watson JD, Dreese JC. Investigation of multiligamentous knee injury patterns with associated injuries presenting at a level I trauma center. J Orthop Trauma 2013;27:226-31. [Crossref] [PubMed]
- Walker REA, McDougall D, Patel S, et al. Radiologic review of knee dislocation: From diagnosis to repair. AJR Am J Roentgenol 2013;201:483-95. [Crossref] [PubMed]
- Gimber LH, Scalcione LR, Rowan A, et al. Multiligamentous injuries and knee dislocations. Skeletal Radiol 2015;44:1559-72. [Crossref] [PubMed]
- Gruber H, Peer S, Meirer R, et al. Peroneal nerve palsy associated with knee luxation: Evaluation by sonography - Initial experiences. AJR Am J Roentgenol 2005;185:1119-25. [Crossref] [PubMed]
- Mattsson P, Alberts A, Dahlberg G, et al. Resorbable cement for the augmentation of internally-fixed unstable trochanteric fractures a prospective, randomised multicentre study. J Bone Joint Surg Br 2005;87:1203-9. [Crossref] [PubMed]
- Werner BC, Gwathmey FW, Higgins ST, et al. Ultra-low velocity knee dislocations: Patient characteristics, complications, and outcomes. Am J Sports Med 2014;42:358-63. [Crossref] [PubMed]
- Stannard JP, Sheils TM, Lopez-Ben RR, et al. Vascular Injuries in Knee Dislocations: The Role of Physical Examination in Determining the Need for Arteriography. J Bone Joint Surg Am 2004;86-A:910-5. [Crossref] [PubMed]
- Natsuhara KM, Yeranosian MG, Cohen JR, et al. What Is the Frequency of Vascular Injury After Knee Dislocation? Clin Orthop Relat Res 2014;472:2615-20. [Crossref] [PubMed]
- McDonough EB, Wojtys EM. Multiligamentous Injuries of the Knee and Associated Vascular Injuries. Am J Sports Med 2009;37:156-9. [Crossref] [PubMed]
- Seroyer ST, Musahl V, Harner CD. Management of the acute knee dislocation: The Pittsburgh experience. Injury 2008;39:710-8. [Crossref] [PubMed]
- Manske RC, Hosseinzadeh P, Giangarra CE. Multiple ligament knee injury: complications. N Am J Sports Phys Ther 2008;3:226-33. [PubMed]
- Lu W, Pauly KB, Gold GE, et al. SEMAC: Slice Encoding for Metal Artifact Correction in MRI. Magn Reson Med 2009;62:66-76. [Crossref] [PubMed]
- Grassi A, Bailey JR, Signorelli C, et al. Magnetic resonance imaging after anterior cruciate ligament reconstruction: A practical guide. World J Orthop 2016;7:638-49. [Crossref] [PubMed]
- Bencardino JT, Beltran J, Feldman MI, et al. MR Imaging of Complications of Anterior Cruciate Ligament Graft Reconstruction. Radiographics 2009;29:2115-26. [Crossref] [PubMed]
- Meyers AB, Haims AH, Menn K, et al. Imaging of Anterior Cruciate Ligament Repair and Its Complications. AJR Am J Roentgenol 2010;194:476-84. [Crossref] [PubMed]
- Recht MP, Kramer J. MR Imaging of the Postoperative Knee: A Pictorial Essay. Radiographics 2002;22:765-74. [Crossref] [PubMed]
- Papakonstantinou O, Chung CB, Chanchairujira K, et al. Complications of anterior cruciate ligament reconstruction: MR imaging. Eur Radiol 2003;13:1106-17. [PubMed]
- Van Dyck P, Lambrecht V, De Smet E, et al. Imaging of the Postoperative Anterior Cruciate Ligament: Emphasis on New Surgical and Imaging Methods. Semin Musculoskelet Radiol 2016;20:33-42. [Crossref] [PubMed]
- Viala P, Marchand P, Lecouvet F, et al. Imaging of the postoperative knee. Diagn Interv Imaging 2016;97:823-37. [Crossref] [PubMed]
- Ilaslan H, Sundaram M, Miniaci A. Imaging evaluation of the postoperative knee ligaments. Eur J Radiol 2005;54:178-88. [Crossref] [PubMed]
- Parkar AP, Alcalá-Galiano A. Rupture of the Posterior Cruciate Ligament: Preoperative and Postoperative Assessment. Semin Musculoskelet Radiol 2016;20:43-51. [Crossref] [PubMed]
- Tsifountoudis I, Karantanas AH. Current Concepts on MRI Evaluation of Postoperative Knee Ligaments. Semin Musculoskelet Radiol 2016;20:74-90. [Crossref] [PubMed]
Cite this article as: Helito PVP, Peters B, Helito CP, Van Dyck P. Imaging evaluation of the multiligament injured knee. Ann Joint 2018;3:80.