Robots in orthopedic surgery
The application of robotics is the most influential achievement of the 20th century (1,2). The earliest robots named Unimate were invented and patented in 1950s (3). And now, the robots can use tools, understand multi-language, drive cars, help nurse elderly people, and can even do hard tasks that cannot be done by manpower (4). It took only 20 years for the robot to learn to walk with two legs from the crawl and become an upright robot while humans spent millions of years crawling to erect (2).
As early as 1985, neurosurgeon performed a stereoscopic proceed with the assistance of a factory robot (5). This was the first time that combined robotic technology with surgeries, which indicated the beginning of an appliance of robotics in medicine (5). After decades of rapid development, medical robots have gained widespread acceptance in many areas. In neurosurgery, the robots are mainly used for precise positioning of brain lesions and for assisting doctors in holding and fixing surgical instruments (6). Laparoscopic robots are used to perform minimally invasive laparoscopic procedures related to cardiac surgery, urology, thoracic surgery, hepatobiliary and pancreatic surgery, gastrointestinal surgery, gynecology and the like (6,7). The core functions of vascular interventional robots are internal propulsion and navigation as well as perception during propelling (7). The robots can also help disable people that without lower limbs to walk and do sports, and help patients who suffered from stroke and nerve injuries to do rehabilitation (8).
As for robotic-assisted orthopedic surgery, the earliest record available was a total hip arthroplasty (THA) by ROBODOC two decades ago (5,9). ROBODOC was subject to approval by the Food and Drug Administration (FDA) of America and was used on patients in 1991 (9). Nowadays, a large number of robotic products have been developed and more orthopedist preferred using a robot for the surgery in order to get a more accurate alignment as well as kinematics. This article is designed to provide an overview on the history of robotics in orthopedic surgery and a review of their current state.
Basic procedure of robot-assisted orthopedic surgery
Virtual modelling
First of all, a sufficiently realistic representation of the patients’ anatomy information will be created by the computer. The surgeons can make the surgery plan according to the virtual model on the screen. Basically, there are two kinds of systems on the basis of the time of image acquisition (10).
In pre-operative image acquisition system, patients’ data is registered before surgery using the preoperative imaging, currently CT or magnetic resonance (MR) imaging, to detail 3-dimensional (3D) images (3). However, it failed to consider that the bony morphology may change after the image acquisition (3,11,12). Using intra-operative image acquisition systems can create a virtual model according to the real-time position after patients lied on the operation tables using intra-operative CT, 2-D, 3-D fluoroscopy or O-arm (10). The traditional way of obtaining images, however, will increase the economic burden and risk of radiation exposure to patients (1,6). Therefore, the radiation-free system, which can record anatomy data by a handed instrument at the surface of surgical objects, was developed. It has more advantages when the tissue structures like ligaments are difficult to identify based on CTs, as the anatomy data is generated intra-operatively by the surgeons (3,10). And the landmarks that cannot directly access can be calculated by the computer (10,12).
The two methods of image acquisition system have their own strengths. The preoperative one can help in positioning the components, simulate alignments of the knee, calculate the angle needed to be corrected, pre-designing the osteotomy plane, and so on. The intraoperative one mainly plays the role of navigation, which can accurately determine the osteotomy plane, however, with a longer operation time.
Registration
Registration, also known as matching, is required if pre-operative images were used for the virtual modeling. The purpose of this step is to match the real position data with the virtual one (10).
A consider number of methodologies have been discovered for matching. In the beginning, pair-point matching is relatively simple, via pairing the feature points on the target object identified by the computer mouse and virtual object identified by the tracked probe (5,6). This method is easy to operation; however, it abandoned the positioning accuracy. A straightforward way is to place a marker on the target tissue before the procedure, which will cause extra wound. Then the calibrated image system, which can provide a registration with a limited number of fluoroscopic images, has been developed. Another registration method is using an ultrasound probe to track the spatial landmarks (10). This method can avoid invasive exposure of the surfaces of the object structures, but the placement of the probe is difficult to prehension, especially when the tissues around the target are thick. The current method mainly using infrared technology, which can provide a precise and accurate measurement of the position information. The intra-operative image acquisition systems do the job of registration while collecting the position information and then create a virtual model (3,10).
Operative procedure
The robot-assisted operation officially begins after matching the real object from the operation table with the virtual one in the computer system. The robotic system can be assigned to three main categories (passive, active and semi-active) based upon the degree of computer control inherent in the methodologies of executions (5,9).
Passive systems like the Acrobat robotic system, need a direct and completely manipulate by the surgeon. For instance, the surgeon manipulates the device but acts on information supplied by the system. While active robotic systems, for example, ROBODOC and iBlock, are able to performing individual tasks autonomously. And semi-active systems, like Navio and MAKO have a degree of autonomy, but still require the surgeon to participant such like define the resection parameters. The robots can help well placed surgical implants and cutting guide plates autonomously, or guide the surgeons for positioning the instruments accurately and efficiently. What’s more, some robotic systems have the function of auto bone milling and cutting.
Specific applications of robot-assisted orthopedic surgery
Arthroplasty
Total joint arthroplasty (TJA) is more suitable for the application of robotic systems because the femur and tibia can be fixed to a specific location in the space (3). There are several commercial systems available for clinical use in TJA.
As the earliest system, ROBODOC has been applied in many countries, the majority of, which was mounted in Germany (5,9). In the early 2000s, nearly 15,000 cases were supported by the system (3,10). It has a robot with five-axis arm equipped who can mill a cavity into the femur for cementless femoral component autonomously. A preoperative fine slice CT scan is mandatory, with fiduciary markers in the distal supracondylar region and the greater trochanter. The new-generation ROBODOC system can use anatomical features to register intraoperative and avoid inserting the fiducial (13). The surgeon then selects optimal implant and accounting for leg length and lateral offsets in ORTHODOC, a 3D computer modeling station. After registration, the surgeon can supervise the autonomous system to mill the cavity of the femur and then implant the femoral component manually. Song et al. compared the range of motion, Western Ontario and McMaster Universities Osteoarthritis Index (WOMAC) scores, and Hospital for Special Surgery (HSS) scores after traditional surgery and robotic-assisted surgery and found no differences in these scores (14,15). Börner and colleagues found the alignment of the knee was 97% consistent with the preoperative planned mechanical axis (16).
CASPAR is an ROBODOC similar system that can autonomously mill bone and preparing the femur with six degrees of freedom (3). Studies have demonstrated an improve tibiofemoral alignment and accuracy in positioning the femoral prosthesis (17,18). However, more limitations were found at the same time. Longer operation time, increased blood loss, and more complications were raised (19). Today, the CASPAR system is not available on the market.
IBlock and Navio PFS were the common two navigation systems. IBlock system uses anatomic data collected intraoperative to provide a 3D digital model of patients’ knee guiding the surgeon to resect with a standard oscillating saw (3,20). Koulalis and colleagues reported more efficient and more accurate femoral cuts using the system comparing with the conventional way (21). Suero et al. demonstrated a significant reduction in variability of mechanical axis and in tourniquet time using iBlock system other than the traditional one (20). And the accuracy and repeatability of bone resections were approved by Ponder and colleagues (22).
Navio PFS, a semi-active system, which approved by FDA in 2012, can track patients’ lower limb position and the degree of knee flexion constantly during the operation (3,23). This imageless system can generate a 3D virtual model of knee and views of the surgical procedure so that the surgeons can gain the real-time limb position and the knee flex degrees during registration and bone preparation. The system is currently only adapted to the unicondylar knee arthroplasty (UKA). By utilizing the Navio PFS, Lonner and colleagues gained a precise implementation of UKA with small errors (23). Although these two systems can provide a decreased cutting phase time, the intra-operative navigation time is still longer than the handed procedure.
Mako is a versatile robotic arm-assisted procedure which is being used commonly for TKA, UKA, and TKA (3,24). It is an imaged-based system, which means CT images are required for figuring the size and position of the component based on the kinematics of patients. As a semi-active system, Mako also can provide intraoperative haptic feedback to avoid exceeding resection from the predetermined area pre-planned based on the preoperative CT (3,5). A considerable number of studies demonstrated an increasing of accuracy and decreasing in a variable in tibial component alignment compared with manual techniques (25-27). The accuracy in achieving desired leg length and offset in THA using Mako system compared with manual instrumentation has been illustrated (28). In TKA, Mako acts as a cutting system that can only use the platform-specific saw blade (3). Mako not only improves the clinical outcomes including patient-reported outcomes, but also decreased the complications and failure rates. Conditt et al. reported a significant higher very satisfied or satisfied rate of patients underwent robotic-assisted UKA procedures at 2-year follow-up (29). Studies demonstrate that even the learning curve of UKA decreased by using Mako robotic system (30). It is currently one of the most popular robotic-assisted system in orthopedic surgery; however, higher requirements will always stimulate an advancement.
Trauma and spine surgery
Computer-assisted navigation system has been involved in the treatment of fractures, providing real-time spatial orientation and depth perception (6). A number of commercial systems have been adapted to the hip fractures as well as long-bone fractures. However, there were still some probabilities of deviation from the planned route been reported, which might result from the system cannot prevent the drill from slipping and deviating from the planned way while the surgery proceeding (3,5,7,9).
Phecda is newly appeared in our sight, as an orthopedic surgical guidance system, it can guide the surgeon to make minimally invasive incisions in spine surgeries and inserts the implants more accurately in multiple fractures (31,32). By using patient-specific images, Phecda can guide the surgeon to the preplanned location to perform required procedures.
Different surgeons may drill different tunnels in cruciate ligament reconstruction, because they mainly base on their experience. However, the robotic system can provide repeatable procedures in different patients depending on the anatomy information. Musahl and colleagues reported an accurate tunnel drilling during CASPAR-assisted anterior cruciate ligament (ACL) reconstruction surgery (33). At present, most researchers are focused on how to increase the precision and accuracy in tunnel placement of cruciate ligament reconstruction with the robot-assisted system. Further research is required to develop a more potential use of the robotic system in treatment of ligamentous knee injuries.
Researchers have demonstrated that robotic systems can help in reducing intraoperative fluoroscopy time and radiation exposure. In spine surgery, the robotic system mainly was used as autonomous pin/screw insertion. MAZOR can combine its devices with the bone for dynamic monitoring, positioning a drill guide whose deviation within 0.2 mm (34,35).
Another system that can autonomously position the end effector to the area of interest, named CRIGOS (9). Although there are no definite clinical studies, in vitro studies reported it can be easily applied in orthopedic surgeries to improve the accuracy and precision.
Discussion
With advances in technology and surgery, orthopedists are chasing for accurate and precise procedures, such as cutting of bone as well as positioning of femoral or tibial components, which are related to the longevity of the components (1,11,12). As shown in Table 1, Bargar et al. found ROBODOC can significantly improve the outcomes of THA in fit, fill, and alignment in contrast with manual work. Jones and colleagues found a visible decrease in postoperative pain and an apparent increase in knee function (5). The robotic devices have gained increasing attention as they can accurately control of cutting and milling within the safety zone without damaging surrounding soft tissues. A multicenter study has reported that the revision rate was as low as 1.2% with the assistant of Mako (3). In addition, the robotic system can also provide a more accurate procedure than conventional techniques when doing tasks like long bone fracture reduction and spinal deformity correction the robots (5,9).
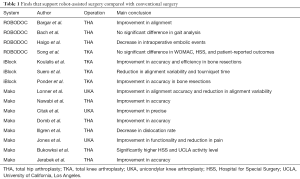
Full table
Furthermore, they are very suitable for young surgeons with less experience to perform operations needed lots experience to achieve the accuracy and precision (10,11). And the results are reproducible, which means that no matter who the surgeon and patient are. The robotic end effector can reduce the variation in outcomes. Besides, the system can provide a 3D model on the screen for the surgeon to do preoperative planning and intraoperative adjustment, either use preoperative or intraoperative information (10). Those models can help surgeons to fully understand the anatomic relationship, especially in patients with severe deformity. What’s more, with the help of virtual reality (VR) technology, junior doctors may receive surgical training without high cost, ethical concerns, and reduction in training hours (36).
Instruments in orthopedic surgeries cost millions of dollars each year (1,3,5). Whether these robots are finally accepted for routine orthopedic procedures, still mainly depend on their cost-effectiveness (11). Robotic devices can help in lowing the instrument costs to manufacturers, because the expensive saw guides, broaches, and reamers will be replaced by cheaper and disposable end effectors (1,6). However, the robot-assisted surgery may not be covered by the medical insurance for using robotic systems in operations routinely is not yet possible as the cost of consumable is relative higher especially when considering the larger population base in China. Moreover, some private hospitals might overuse these systems in order to obtain benefits and recover the cost of buying surgical robots.
Although the application of robotic systems in surgeries seemed to have certain advantages, there several issues needed to address. First of all, it is necessary to address the issue of safe use of robotic devices in the operating room (OR). Although many studies found it’s safe to use robot-assisted devices in operations. Some literatures illustrated that all personnel must be well-trained and standard assessment, and the regularized process flow must be established (11). The second issue is the time cost. These robotic procedures can provide accurate and precise outcomes. However, literature demonstrated that time spending in OR increased 30 minutes averagely using the ROBODOC system, which will result in an increased risk of associated complications (5). In the near feature, the dilatory motion of robotic devices may be improved in a more efficient way. Thirdly, various studies declared that there was no difference in clinical and radiological outcomes compared robot-assisted surgeries with conventional ones. Others believed that the success or failure of using robotic systems still needs long-term randomized clinical trials to prove.
Today, the robotic technology is in the process of being state-of-the-art for orthopedic use since it emerged in the last century (6). Despite some minor issues, the benefits are clear. We believe that, in time, robotic devices will be the routine equipment of orthopedic procedure.
Acknowledgments
Funding: This study was supported by the International Cooperation and Exchanges NSFC (81420108021), Key Program of NSFC (81730067), Excellent Young Scholars NSFC (81622033), NSFC (51575100, 51705259), Jiangsu Provincial Key Medical Center Foundation and Jiangsu Provincial Medical Outstanding Talent Foundation.
Footnote
Conflicts of Interest: All authors have completed the ICMJE uniform disclosure form (available at http://dx.doi.org/10.21037/aoj.2018.02.01). DS serves as an unpaid Executive Editor-in-Chief of Annals of Joint from Mar 2016 to Feb 2021. QJ serves as an Editor-in-Chief of Annals of Joint from Mar 2016 to Feb 2021. The other authors have no conflicts of interest to declare.
Ethical Statement: The authors are accountable for all aspects of the work in ensuring that questions related to the accuracy or integrity of any part of the work are appropriately investigated and resolved.
Open Access Statement: This is an Open Access article distributed in accordance with the Creative Commons Attribution-NonCommercial-NoDerivs 4.0 International License (CC BY-NC-ND 4.0), which permits the non-commercial replication and distribution of the article with the strict proviso that no changes or edits are made and the original work is properly cited (including links to both the formal publication through the relevant DOI and the license). See: https://creativecommons.org/licenses/by-nc-nd/4.0/.
References
- Beasley RA. Medical Robots: Current Systems and Research Directions. Journal of Robotics 2012;2012:1-14.
- Cai Z. Robotics. Beijing: Tsinghua University Press, 2000:1-7.
- Jacofsky DJ, Allen M. Robotics in Arthroplasty: A Comprehensive Review. J Arthroplasty 2016;31:2353-63. [Crossref] [PubMed]
- Ni Z, Wang T, Liu D. Survey on Medical Robotics. J Mech Eng 2015;51:45-52. [Crossref]
- Bargar WL. Robots in orthopaedic surgery: past, present, and future. Clin Orthop Relat Res 2007;31-6. [PubMed]
- Cleary K, Nguyen C. State of the Art in Surgical Robotics: Clinical Applications and Technology Challenges. Comput Aided Surg 2001;6:312-28. [Crossref] [PubMed]
- Pott P, Schwarz M. Robots, navigation, telesurgery: state of the art and market overview. Z Orthop Ihre Grenzgeb 2002;140:218-31. [Crossref] [PubMed]
- Hussain S, Jamwal PK, Ghayesh MH. Single joint robotic orthoses for gait rehabilitation: An educational technical review. J Rehabil Med 2016;48:333-8. [Crossref] [PubMed]
- Adili A. Robot-assisted orthopedic surgery. Semin Laparosc Surg 2004;11:89-98. [PubMed]
- Zheng G, Nolte LP. Computer-Assisted Orthopedic Surgery: Current State and Future Perspective. Front Surg 2015;2:66. [Crossref] [PubMed]
- Díaz CE, Fernandez R, Armada M, et al. A research review on clinical needs, technical requirements, and normativity in the design of surgical robots. Int J Med Robot 2017;13:e1801 [Crossref] [PubMed]
- Schneider J, Kalender W. Geometric Accuracy in Robot-Assisted Total Hip Replacement Surgery. Comput Aided Surg 2003;8:135-45. [Crossref] [PubMed]
- Liow MHL, Chin PL, Pang HN, et al. THINK surgical TSolution-One(®) (Robodoc) total knee arthroplasty. SICOT J 2017;3:63. [Crossref] [PubMed]
- Song EK, Seon JK, Park SJ, et al. Simultaneous bilateral total knee arthroplasty with robotic and conventional techniques: a prospective, randomized study. Knee Surg Sports Traumatol Arthrosc 2011;19:1069-76. [Crossref] [PubMed]
- Song EK, Seon JK, Yim JH, et al. Robotic-assisted TKA Reduces Postoperative Alignment Outliers and Improves Gap Balance Compared to Conventional TKA. Clin Orthop Relat Res 2013;471:118-26. [Crossref] [PubMed]
- Börner M, Wiesel U, Ditzen W. Clinical Experiences with ROBODOC and the Duracon Total Knee. Navigation and Robotics in Total Joint and Spine Surgery. Berlin, Heidelberg: Springer Berlin Heidelberg, 2004:362-6.
- Siebert W, Mai S, Kober R, et al. Technique and first clinical results of robot-assisted total knee replacement. Knee 2002;9:173-80. [Crossref] [PubMed]
- Wu LD, Hahne HJ, Hassenpflug J. The dimensional accuracy of preparation of femoral cavity in cementless total hip arthroplasty. J Zhejiang Univ Sci 2004;5:1270-8. [Crossref] [PubMed]
- Siebel T, Käfer W. Klinisches Outcome nach Roboter-assistierter versus konventionell implantierter Hüftendoprothetik: Prospektive, kontrollierte Untersuchung von 71 Patienten. Z Orthop Ihre Grenzgeb 2005;143:391-8. [Crossref] [PubMed]
- Suero EM, Plaskos C, Dixon PL, et al. Adjustable cutting blocks improve alignment and surgical time in computer-assisted total knee replacement. Knee Surg Sports Traumatol Arthrosc 2012;20:1736-41. [Crossref] [PubMed]
- Koulalis D, O'Loughlin PF, Plaskos C, et al. Sequential versus automated cutting guides in computer-assisted total knee arthroplasty. Knee 2011;18:436-42. [Crossref] [PubMed]
- Ponder CE, Plaskos C, Cheal EJ. Press-fit total knee arthroplasty with a robotic-cutting guide: proof of concept and initial clinical experience. Bone Jt J 2013;95-B:61.
- Lonner JH, Smith JR, Picard F, et al. High Degree of Accuracy of a Novel Image-free Handheld Robot for Unicondylar Knee Arthroplasty in a Cadaveric Study. Clin Orthop Relat Res 2015;473:206-12. [Crossref] [PubMed]
- Tamam C, Poehling GG. Robotic-assisted unicompartmental knee arthroplasty. Sports Med Arthrosc Rev 2014;22:219-22. [Crossref] [PubMed]
- Lonner JH. Indications for unicompartmental knee arthroplasty and rationale for robotic arm-assisted technology. Am J Orthop (Belle Mead NJ) 2009;38:3-6. [PubMed]
- Sinha RK. Outcomes of robotic arm-assisted unicompartmental knee arthroplasty. Am J Orthop (Belle Mead NJ) 2009;38:20-2. [PubMed]
- Pearle AD, O'Loughlin PF, Kendoff DO. Robot-Assisted Unicompartmental Knee Arthroplasty. J Arthroplasty 2010;25:230-7. [Crossref] [PubMed]
- Nawabi DH, Conditt MA, Ranawat AS, et al. Haptically guided robotic technology in total hip arthroplasty: a cadaveric investigation. Proc Inst Mech Eng H 2013;227:302-9. [Crossref] [PubMed]
- Conditt MA, Coon T, Hernandez A, et al. Short term survivorship and out- comes of robotically assisted bicompartmental arthroplasty. Bone Joint J 2016;98-B:49. [PubMed]
- Hamilton WG, Ammeen D, Engh CA, et al. Learning curve with minimally invasive unicompartmental knee arthroplasty. J Arthroplasty 2010;25:735-40. [Crossref] [PubMed]
-
. Available online: http://www.tinavi.com/index.php?m=content&c=index&a=lists&catid=25Products and Support of Intelligent Medical Solutions - Tian W, Wang H, Liu YJ. Robot-assisted Anterior Odontoid Screw Fixation: A Case Report. Orthop Surg 2016;8:400-4. [Crossref] [PubMed]
- Musahl V, Burkart A, Debski RE, et al. Accuracy of anterior cruciate ligament tunnel placement with an active robotic system: A cadaveric study. Arthroscopy 2002;18:968-73. [Crossref] [PubMed]
- Wolf A, Shoham M, Michael S, et al. Feasibility study of a mini, bone-attached, robotic system for spinal operations: analysis and experiments. Spine 2004;29:220-8. [Crossref] [PubMed]
- Sukovich W, Brink-Danan S, Hardenbrook M. Miniature robotic guidance for pedicle screw placement in posterior spinal fusion: early clinical experience with the SpineAssist. Int J Med Robot 2006;2:114-22. [Crossref] [PubMed]
- Li L, Yu F, Shi D, et al. Application of virtual reality technology in clinical medicine. Am J Transl Res 2017;9:3867-80. [PubMed]
Cite this article as: Yu F, Li L, Teng H, Shi D, Jiang Q. Robots in orthopedic surgery. Ann Joint 2018;3:15.