Bony morphologic factors affecting injury risk, rotatory stability, outcomes, and re-tear rate after anterior cruciate ligament reconstruction
Introduction
Anterior cruciate ligament (ACL) reconstruction is an increasingly common orthopaedic procedure (1,2). Outcomes after ACL reconstruction have improved considerably as surgical technique, implant and imaging technology, and scientific understanding have advanced over the past few decades of modern ACL reconstruction. However, there remains a subset of patients that have subpar outcomes after ACL reconstruction such as residual rotatory instability, graft re-tear, and premature arthritis (3-5). The underlying etiology for these unsatisfactory outcomes is multifactorial, and anatomic, biologic, neuromuscular, and technical factors all play a role (6-12). Recently, several studies have demonstrated that certain bony characteristics of the patient’s native anatomy may contribute to rotatory knee stability (Table 1) (13-17). As surgeons strive to improve treatment of ACL injuries, knowledge of these bony factors which affect knee stability is crucial to optimize patient outcomes.
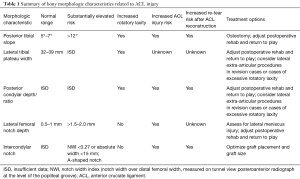
Full table
Tibial slope
The unique shapes of the medial and lateral tibial plateaus contribute to the characteristic rotatory instability associated with ACL injury. The lateral tibial plateau is convex and has a smaller surface area than the medial plateau. The complex interaction of the lateral tibial plateau and lateral femoral condyle leads to the prototypical pivot shift sign, or “clunk”, observed in the setting of ACL deficiency. In fact, the slope of the lateral tibial plateau plays an important role in rotatory knee stability and ACL re-injury risk (13,18-21). For instance, a study of 11 female cadaveric knees reported a mean lateral tibial slope of 7.6 degrees, and the authors reported that increasing the tibial slope was significantly associated with anterior tibial acceleration and ACL strain during dynamic impact testing (21).
The effect of tibial slope on rotatory knee stability and injury risk have also been observed in clinical studies. For instance, one study demonstrated that ACL tear patients with high grade rotatory laxity have a higher tibial slope than those with low grade rotatory laxity (13). A separate study of 200 consecutive patients who underwent ACL reconstruction reported a mean tibial slope of 8.5 degrees, although patients who had sustained a second ACL injury at 15-year follow-up had a tibial slope of 9.9 degrees (P=0.001) (22). Hashemi et al. demonstrated that for each degree increase in lateral tibial slope, ACL injury risk increased by 1.17-fold (23). In a separate study comparing 88 ACL reconstruction patients to 88 control population, authors reported a 21.7% increased risk of noncontact ACL injury with each degree of increased slope in females, but not males (20). However, other studies have reported increased injury risk in males with increased tibial slopes. In fact, a recent study in division-1 collegiate football athletes found that lateral tibial slope predicted ACL injury risk (19). Unpublished data from our institution has shown that increased tibial slope (Figure 1) leads to altered gait kinematics and tibial rotation during downhill running. As demonstrated by the previous studies, increased lateral compartment tibial slope results in increased rotatory knee laxity, ACL strain, and ultimately ACL injury risk.
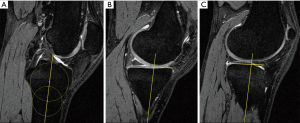
Tibial plateau size
Musahl et al. studied size parameters of the femoral condyle and tibial plateau. The authors studied a total of 49 patients (males and females). They performed pivot shift testing in patients with ACL tears under anesthesia. The authors reported that patients with a smaller lateral tibial plateau diameter in the medial to lateral direction also had higher grade pivot shifts. When analyzed by sex, this applied to females, but not males. They did not find associations between other morphologic parameters and pivot shift grade. The authors originally hypothesized that the anterior to posterior dimensions of the tibia would be most associated with rotatory stability. They explained the discrepancy between the original hypothesis and their finding by reasoning that the orientation of the tibia relative to the femoral condyle changes during the pivot shift mechanism, and the medial to lateral dimensions are likely oriented in more of an anterior to posterior direction during this phenomenon (24).
Posterior condylar offset
While distal femoral posterior condylar dimensions have long been discussed in joint arthroplasty literature (25), this specific anatomic feature has just recently been studied in the setting of ACL injury (26). In a study of in vivo knee kinematics using dynamic stereo radiographs and 3D CT scans, a higher posterior condylar offset ratio (COR) was positively correlated with increased anterior tibial translation. A cadaveric study reported a link between posterior condylar offset and posterior tibial slope (27). In ongoing but unpublished research at our institution, increased posterior condylar depth (Figure 2), relative to overall condyle depth in the anterior to posterior direction, as measured on lateral radiographs, has been correlated with an increased risk of non-contact ACL injury and re-injury after ACL reconstruction. Furthermore, quantitative pivot shift analysis of patients with ACL tears utilizing video image analysis software and inertial sensors (28) demonstrated increased rotatory knee laxity in patients with high posterior condylar offset ratios. The mechanism behind the increased knee laxity and injury risk are currently unknown. It is possible that the increased condylar offset results in more of a stretch of the lateral tissues and relative low density when the knee is at lower flexion angles, such as those commonly encountered in non-contact ACL injury. On the other hand, perhaps the injuries occur when the knee is in greater degrees of flexion and the lateral soft tissue structures are at maximum tension and therefore are most likely to fail. Studies have suggested that femoral condyle geometry is important in rotatory stability (29), though future studies will be needed to better understand this relationship.
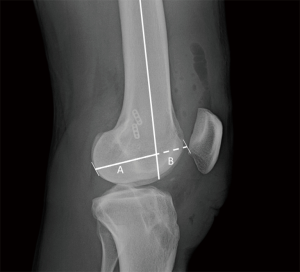
Lateral femoral notch sign
The lateral femoral notch sign (LFN) sign has long been recognized as an indicator of ACL injury. The LFN occurs close to the location of the native condylopatellar sulcus (Figure 3). Supraphysiologic depth and length of this condylopatellar sulcus, as measured on lateral radiographs or sagittal MRI, is referred to as “positive notch sign”. In 1992, Cobby et al. reported that patients with normal ACLs had a LFN depth of 0.45 mm on lateral radiographs, while patients with torn ACL’s had LFN depth of 0.89 mm. The authors considered LFN depth of greater than 1.5 mm to be a sign of ACL injury. A later MRI study observed that 83% of acute ACL patients had an osseous contusion at the area of the sulcus, and that most involved injury to the subchondral bone (30). Gimberg et al. confirmed that the LFN was associated with ACL injury and further reported that a “double notch” sign was 17% sensitive and 100% specific for complete ACL tear (31).
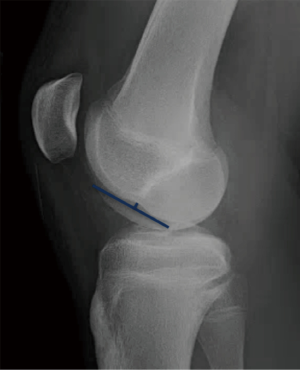
More recently, Herbst et al. reviewed MRIs and X-rays for 500 acute arthroscopically confirmed ACL tears. They observed that ACL tears sustained during high intensity cutting sports were more likely to be accompanied by a deep lateral femoral notch (>2.0 mm) than non-cutting sports. They also found a significant correlation between LFN >2.0 mm and presence of a lateral meniscus tear (15). Recent research from the authors’ institution has corroborated the association between LFN depth and lateral meniscal tears. However, there was no significant correlation between LFN depth and quantitative pivot shift measurements. While the relationship between LFN depth and rotatory injuries such as ACL and lateral meniscal tears is clear, more research is needed to elucidate the pathogenesis of the LFN.
Intercondylar notch
Perhaps no bony morphologic feature has been more studied in the context of ACL injury than the intercondylar notch (Figure 4). In fact, a PubMed search in June 2017 reveals over 350 articles on the topic of intercondylar notch and ACL. As early as 1983, the intercondylar notch was implicated in ACL injury (32,33). Subsequently in 1987, Houseworth et al. used computer graphic analysis to suggest that narrow notch width may predispose the individual to ACL injury (34). Then in 1988, Souryal et al. reported that individuals with bilateral ACL tears had significantly narrower notches than a control group (35). The authors also described the measurement of the notch width index (NWI), which was the ratio of the width of the intercondylar notch relative to the notch of the distal femur, measured at the level of the popliteal groove on posteroanterior “tunnel view” radiographs. A separate study performed by the same group reported that high school athletes sustaining noncontact ACL injuries had lower NWI than the uninjured cohort (36). The relationship between narrow notch width and increased ACL injury risk has been confirmed not just in young patients with acute ACL tears, but also in older patients with knee arthritis (37). In general, an NWI of <0.27 or an absolute notch width of <15 mm is considered a significant risk factor for ACL injury (38).
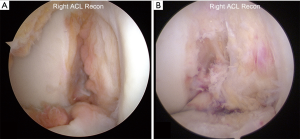
Since these early studies, our understanding of the intercondylar notch has continued to evolve. Studies have shown that a smaller notch size often correlates with a smaller ACL, suggesting that the increased injury risk could partially be due to a weaker ACL (39-41). Other studies characterized the various shapes of notches, and linked “A-shaped” notches to increased risk of ACL injury (16,42). Multiple research articles have analyzed notch differences among various populations. For instance, some studies reported that females have narrower notches, a higher proportion of A-shaped notches, and less notch volume than their male counterparts (16,41-43). Similarly, Caucasian males and females were found to have narrower notches than African American men (44). In both cases, the populations with characteristic notch morphology have correspondingly higher risks of non-contact ACL injury.
Using cadaveric specimens, robotic loading and 3-D mathematical models, Fung et al. demonstrated the impingement of the ACL against the intercondylar notch. They determined that slight knee flexion, tibial external rotation, and knee abduction resulted in the greatest degree of impingement. The authors noted that although tibial internal rotation results in direct ACL strain, it decreases the distance to the notch wall and thus lowers the risk of impingement (45). Park et al. followed up on this earlier work and expounded upon its findings. They reported that ACL impingement was first detected at 15° of tibial external rotation and 4° of abduction. Further abduction and external rotation increased the impingement force. In fact, the model showed that 29° of external rotation and 10° of abduction resulted in ACL stretching and deformation (46). An animal model showed that repetitive impingement of the ACL in this position of tibial external rotation and abduction resulted in cell death and cumulative ligament damage (47), and more recent studies have corroborated this mechanism of notch impingement (Figure 5) (48).
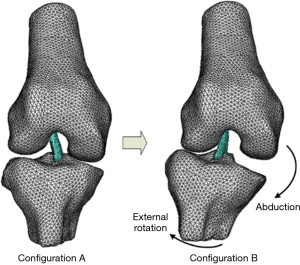
This knowledge of notch morphology should encourage thorough assessment of the notch during preoperative planning and during the surgical procedure. In addition to the relationship between narrower notches and smaller native ACLs, studies have shown that larger grafts used during ACL reconstruction could lead to increased impingement on the notch (49). In fact, ongoing research at our own institution has demonstrated that increased graft size relative to notch width at the apex can result in increased failure rate. This is important as most of the literature to date on graft size has focused on risk of re-tear risk when smaller grafts have been used (50).
Graft positioning also influences the risk of graft impingement. Non-anatomic placement of the femoral tunnel has been cited as the leading technical cause of ACL reconstruction failure (51,52). Several studies have demonstrated that high and anterior placement of the femoral tunnel can result in increased impingement against the notch (49,53). Similarly, anterior and lateral positioning of the tibial tunnel results in increased graft impingement (49,53,54). As such, surgeons should consider tibial tunnel-independent methods of femoral tunnel placement, such as anteromedial portal or outside-in drilling (55). These techniques have demonstrated more anatomic positioning of both the tibial and femoral tunnels, and thus decreasing the risk of graft impingement (56-60).
Discussion
As demonstrated above, a variety of bony morphologic factors can impact the risk of primary or repeat ACL injury. Some of these can be addressed surgically, and others are merely harbingers of ACL injury or rotatory laxity. Some of these bony characteristics are part of the normal developmental process, while others result from injury, and still others may develop as a result of chronic microtrauma or altered gait patterns. Wolff hypothesized the relationship between bone structure and function as early as 1867 (61). In fact, Wolff’s law has remained a cornerstone in modern understanding of bone and its dynamic responses to pressure, tension, and its surrounding environment (62,63).
While the Mechanostat model of bone has been known for nearly 150 years, and has been studied in-depth in other settings, the study of bone morphology and its interaction with ACL function is in a stage of relative infancy. McNeill Ingham, Lovejoy, and others have provided crucial insight into the evolutionary development of the human distal femur, and its comparison to other bipedal species (64). For instance, cows have demonstrated a similar NWI to humans, while goats, dogs, and other animals did not. However, pigs showed sex-specific differences in notch morphology that mirrored that of the human differences in male and female notches. Furthermore, most quadrupeds have a steeper tibial slope and narrower intercondylar notch relative to bipedal species such as humans. Spending more time in deep knee flexion, and thus necessitating different demands on their knee joints than that of humans, quadrupeds have different knee morphology that is more advantageous to their functional demands (64).
Until recently, bony morphology has been underappreciated in the field of ACL research. In fact, there is still much that is unknown about the causes, consequences, and variations in bony morphology relative to ACL injury. It is crucial that surgeons and scientists continue their pursuit of knowledge and understanding of these factors. As there exists great variability among individuals, incorporation of bony morphology into preoperative planning, operative techniques, and postoperative rehabilitation and return-to-play will be crucial to providing individualized treatment of ACL injuries. For example, in patients with an A-shaped or narrow notch, graft size and positioning must be performed with meticulous attention to detail to ensure that the risk of graft impingement is minimized. In patients with excessive LFN depth, surgeons should assess the preoperative imaging and intraoperative exam for lateral meniscus injuries that might otherwise be missed. Preoperative x-rays with a deep LFN should alert clinicians of a likely ACL injury in the absence of an MRI or with equivocal MRI findings. Patients with excessive posterior tibial slope or posterior condylar offset/depth should be considered to have higher rotatory laxity and increased re-injury risk. Tibial slope can be addressed with a slope-reducing osteotomy, especially during revision ACL reconstruction (65). Although no surgical treatments are known to address the distal femoral condylar anatomy, surgeons may consider additional procedures such as extra-articular tenodesis or more conservative postoperative protocols.
Conclusions
In conclusion, numerous bony morphologic features have shown association with ACL injury risk, rotatory knee laxity, and outcomes after ACL reconstruction. Posterior tibial slope and posterior femoral condyle characteristics have been correlated to measurements of rotatory knee stability, with increasing slope and greater condylar depth resulting in greater knee laxity. Increased tibial slope, increased posterior femoral condylar depth, a deepened lateral femoral notch (condylopatellar sulcus), and smaller intercondylar notch morphology have demonstrated increased ACL injury risk, and in some cases ACL reconstruction failure rates. Although historically underappreciated, it is important that surgeons consider these individual anatomic bony features when treating patients with ACL injury. Furthermore, future research should continue to focus on elucidating the pathogenesis behind abnormal bony morphology and its relationship with ACL injury risk. ACL reconstruction is not a one-sized fitting all surgery, and understanding the impact of subtle bony morphologic variations will guide surgeons to deliver more individualized treatment options.
Acknowledgments
Funding: None.
Footnote
Provenance and Peer Review: This article was commissioned by the editorial office, Annals of Joint for the series “Trends in ACL Reconstruction”. The article has undergone external peer review.
Conflicts of Interest: All authors have completed the ICMJE uniform disclosure form (available at http://dx.doi.org/10.21037/aoj.2017.06.11). The series “Trends in ACL Reconstruction” was commissioned by the editorial office without any funding or sponsorship. JMB served as the unpaid Guest Editor of the series. FFH served as the unpaid Guest Editor of the series and serves as Editor-in-Chief of Annals of Joint from Mar 2016 to Aug 2019. The authors have no other conflicts of interest to declare.
Ethical Statement: The authors are accountable for all aspects of the work in ensuring that questions related to the accuracy or integrity of any part of the work are appropriately investigated and resolved.
Open Access Statement: This is an Open Access article distributed in accordance with the Creative Commons Attribution-NonCommercial-NoDerivs 4.0 International License (CC BY-NC-ND 4.0), which permits the non-commercial replication and distribution of the article with the strict proviso that no changes or edits are made and the original work is properly cited (including links to both the formal publication through the relevant DOI and the license). See: https://creativecommons.org/licenses/by-nc-nd/4.0/.
References
- Mall NA, Abrams GD, Azar FM, et al. Trends in primary and revision anterior cruciate ligament reconstruction among National Basketball Association team physicians. Am J Orthop (Belle Mead NJ) 2014;43:267-71. [PubMed]
- Mall NA, Chalmers PN, Moric M, et al. Incidence and trends of anterior cruciate ligament reconstruction in the United States. Am J Sports Med 2014;42:2363-70. [Crossref] [PubMed]
- Musahl V, Getgood A, Neyret P, et al. Contributions of the anterolateral complex and the anterolateral ligament to rotatory knee stability in the setting of ACL Injury: a roundtable discussion. Knee Surg Sports Traumatol Arthrosc 2017;25:997-1008. [Crossref] [PubMed]
- Vacek PM, Slauterbeck JR, Tourville TW, et al. Multivariate Analysis of the Risk Factors for First-Time Noncontact ACL Injury in High School and College Athletes: A Prospective Cohort Study With a Nested, Matched Case-Control Analysis. Am J Sports Med 2016;44:1492-501. [Crossref] [PubMed]
- Joreitz R, Lynch A, Rabuck S, et al. Patient-Specific and Surgery-Specific Factors That Affect Return to Sport after Acl Reconstruction. Int J Sports Phys Ther 2016;11:264-78. [PubMed]
- Zebis MK, Andersen CH, Bencke J, et al. Neuromuscular Coordination Deficit Persists 12 Months after ACL Reconstruction But Can Be Modulated by 6 Weeks of Kettlebell Training: A Case Study in Women's Elite Soccer. Case Rep Orthop 2017;2017:4269575
- Rahnemai-Azar AA, Zlotnicki J, Burnham JM, et al. Secondary Stabilizers of the Anterior Cruciate Ligament—Deficient Knee. Oper Tech Orthop 2017; [Epub ahead of print]. [Crossref]
- Noyes FR, Huser LE, Levy MS. Rotational Knee Instability in ACL-Deficient Knees: Role of the Anterolateral Ligament and Iliotibial Band as Defined by Tibiofemoral Compartment Translations and Rotations. J Bone Joint Surg Am 2017;99:305-14. [Crossref] [PubMed]
- Tavlo M, Eljaja S, Jensen JT, et al. The role of the anterolateral ligament in ACL insufficient and reconstructed knees on rotatory stability: A biomechanical study on human cadavers. Scand J Med Sci Sports 2016;26:960-6. [Crossref] [PubMed]
- Signorelli C, Filardo G, Bonanzinga T, et al. ACL rupture and joint laxity progression: a quantitative in vivo analysis. Knee Surg Sports Traumatol Arthrosc 2016;24:3605-11. [Crossref] [PubMed]
- Kittl C, El-Daou H, Athwal KK, et al. The Role of the Anterolateral Structures and the ACL in Controlling Laxity of the Intact and ACL-Deficient Knee. Am J Sports Med 2016;44:345-54. [Crossref] [PubMed]
- Fältström A, Hagglund M, Magnusson H, et al. Predictors for additional anterior cruciate ligament reconstruction: data from the Swedish national ACL register. Knee Surg Sports Traumatol Arthrosc 2016;24:885-94. [Crossref] [PubMed]
- Rahnemai-Azar AA, Abebe ES, Johnson P, et al. Increased lateral tibial slope predicts high-grade rotatory knee laxity pre-operatively in ACL reconstruction. Knee Surg Sports Traumatol Arthrosc 2017;25:1170-6. [Crossref] [PubMed]
- Hoffelner T, Pichler I, Moroder P, et al. Segmentation of the lateral femoral notch sign with MRI using a new measurement technique. BMC Musculoskelet Disord 2015;16:217. [Crossref] [PubMed]
- Herbst E, Hoser C, Tecklenburg K, et al. The lateral femoral notch sign following ACL injury: frequency, morphology and relation to meniscal injury and sports activity. Knee Surg Sports Traumatol Arthrosc 2015;23:2250-8. [Crossref] [PubMed]
- van Eck CF, Martins CA, Vyas SM, et al. Femoral intercondylar notch shape and dimensions in ACL-injured patients. Knee Surg Sports Traumatol Arthrosc 2010;18:1257-62. [Crossref] [PubMed]
- Farrow LD, Chen MR, Cooperman DR, et al. Morphology of the femoral intercondylar notch. J Bone Joint Surg Am 2007;89:2150-5. [PubMed]
- Bojicic KM, Beaulieu ML, Imaizumi Krieger DY, et al. Association Between Lateral Posterior Tibial Slope, Body Mass Index, and ACL Injury Risk. Orthop J Sports Med 2017;5:2325967116688664 [Crossref] [PubMed]
- Rahnemai-Azar AA, Yaseen Z, van Eck CF, et al. Increased Lateral Tibial Plateau Slope Predisposes Male College Football Players to Anterior Cruciate Ligament Injury. J Bone Joint Surg Am 2016;98:1001-6. [Crossref] [PubMed]
- Beynnon BD, Hall JS, Sturnick DR, et al. Increased slope of the lateral tibial plateau subchondral bone is associated with greater risk of noncontact ACL injury in females but not in males: a prospective cohort study with a nested, matched case-control analysis. Am J Sports Med 2014;42:1039-48. [Crossref] [PubMed]
- McLean SG, Oh YK, Palmer ML, et al. The relationship between anterior tibial acceleration, tibial slope, and ACL strain during a simulated jump landing task. J Bone Joint Surg Am 2011;93:1310-7. [Crossref] [PubMed]
- Webb JM, Salmon LJ, Leclerc E, et al. Posterior tibial slope and further anterior cruciate ligament injuries in the anterior cruciate ligament-reconstructed patient. Am J Sports Med 2013;41:2800-4. [Crossref] [PubMed]
- Hashemi J, Chandrashekar N, Mansouri H, et al. Shallow medial tibial plateau and steep medial and lateral tibial slopes: new risk factors for anterior cruciate ligament injuries. Am J Sports Med 2010;38:54-62. [Crossref] [PubMed]
- Musahl V, Ayeni OR, Citak M, et al. The influence of bony morphology on the magnitude of the pivot shift. Knee Surg Sports Traumatol Arthrosc 2010;18:1232-8. [Crossref] [PubMed]
- Malviya A, Lingard E, Weir D, et al. Predicting range of movement after knee replacement: the importance of posterior condylar offset and tibial slope. Knee Surg Sports Traumatol Arthrosc 2009;17:491-8. [Crossref] [PubMed]
- Hoshino Y, Wang JH, Lorenz S, et al. The effect of distal femur bony morphology on in vivo knee translational and rotational kinematics. Knee Surg Sports Traumatol Arthrosc 2012;20:1331-8. [Crossref] [PubMed]
- Weinberg DS, Gebhart JJ, Wera GD. An Anatomic Investigation Into the Relationship Between Posterior Condylar Offset and Posterior Tibial Slope of One Thousand One Hundred Thirty-Eight Cadaveric Knees. J Arthroplasty 2017;32:1659-1664.e1. [Crossref] [PubMed]
- Musahl V, Griffith C, Irrgang JJ, et al. Validation of Quantitative Measures of Rotatory Knee Laxity. Am J Sports Med 2016;44:2393-8. [Crossref] [PubMed]
- Fridén T, Jonsson A, Erlandsson T, et al. Effect of femoral condyle configuration on disability after an anterior cruciate ligament rupture. 100 patients followed for 5 years. Acta Orthop Scand 1993;64:571-4. [Crossref] [PubMed]
- Speer KP, Spritzer CE, Bassett FH 3rd, et al. Osseous injury associated with acute tears of the anterior cruciate ligament. Am J Sports Med 1992;20:382-9. [Crossref] [PubMed]
- Grimberg A, Shirazian H, Torshizy H, et al. Deep lateral notch sign and double notch sign in complete tears of the anterior cruciate ligament: MR imaging evaluation. Skeletal Radiol 2015;44:385-91. [Crossref] [PubMed]
- Kieffer DA, Curnow RJ, Southwell RB, et al. Anterior cruciate ligament arthroplasty. Am J Sports Med 1984;12:301-12. [Crossref] [PubMed]
- Lambert KL. Vascularized patellar tendon graft with rigid internal fixation for anterior cruciate ligament insufficiency. Clin Orthop Relat Res 1983;85-9. [PubMed]
- Houseworth SW, Mauro VJ, Mellon BA, et al. The intercondylar notch in acute tears of the anterior cruciate ligament: a computer graphics study. Am J Sports Med 1987;15:221-4. [Crossref] [PubMed]
- Souryal TO, Moore HA, Evans JP. Bilaterality in anterior cruciate ligament injuries: associated intercondylar notch stenosis. Am J Sports Med 1988;16:449-54. [Crossref] [PubMed]
- Souryal TO, Freeman TR. Intercondylar notch size and anterior cruciate ligament injuries in athletes. A prospective study. Am J Sports Med 1993;21:535-9. [Crossref] [PubMed]
- Stein V, Li L, Guermazi A, et al. The relation of femoral notch stenosis to ACL tears in persons with knee osteoarthritis. Osteoarthritis Cartilage 2010;18:192-9. [Crossref] [PubMed]
- Wolf MR, Murawski CD, van Diek FM, et al. Intercondylar notch dimensions and graft failure after single- and double-bundle anterior cruciate ligament reconstruction. Knee Surg Sports Traumatol Arthrosc 2015;23:680-6. [Crossref] [PubMed]
- Chandrashekar N, Slauterbeck J, Hashemi J. Sex-based differences in the anthropometric characteristics of the anterior cruciate ligament and its relation to intercondylar notch geometry: a cadaveric study. Am J Sports Med 2005;33:1492-8. [Crossref] [PubMed]
- Wolters F, Vrooijink SH, Van Eck CF, et al. Does notch size predict ACL insertion site size? Knee Surg Sports Traumatol Arthrosc 2011;19:S17-21. [Crossref] [PubMed]
- Dienst M, Schneider G, Altmeyer K, et al. Correlation of intercondylar notch cross sections to the ACL size: a high resolution MR tomographic in vivo analysis. Arch Orthop Trauma Surg 2007;127:253-60. [Crossref] [PubMed]
- Ireland ML, Ballantyne BT, Little K, et al. A radiographic analysis of the relationship between the size and shape of the intercondylar notch and anterior cruciate ligament injury. Knee Surg Sports Traumatol Arthrosc 2001;9:200-5. [Crossref] [PubMed]
- Wratten CJ, Tetsworth K, Hohmann E. Three-Dimensional Femoral Notch Volume in Anterior Cruciate Ligament-Deficient Versus Anterior Cruciate Ligament-Intact Patients: A Matched Case-Control Study With Inter-gender Comparison. Arthroscopy 2015;31:1117-22. [Crossref] [PubMed]
- Shelbourne KD, Gray T, Benner RW. Intercondylar notch width measurement differences between African American and white men and women with intact anterior cruciate ligament knees. Am J Sports Med 2007;35:1304-7. [Crossref] [PubMed]
- Fung DT, Zhang LQ. Modeling of ACL impingement against the intercondylar notch. Clin Biomech (Bristol, Avon) 2003;18:933-41. [Crossref] [PubMed]
- Park HS, Ahn C, Fung DT, et al. A knee-specific finite element analysis of the human anterior cruciate ligament impingement against the femoral intercondylar notch. J Biomech 2010;43:2039-42. [Crossref] [PubMed]
- Fung DT, Quinby JS, Koh JL, et al. Changes in the rat ACL resulting from subfailure impingement loading. Proceedings of XXth Congress of the International Society of Biomechanics and 29th Annual Meeting of the American Society of Biomechanics. Cleveland, OH, 2005.
- Branch TP, Browne JE, Campbell JD, et al. Rotational laxity greater in patients with contralateral anterior cruciate ligament injury than healthy volunteers. Knee Surg Sports Traumatol Arthrosc 2010;18:1379-84. [Crossref] [PubMed]
- Orsi AD, Canavan PK, Vaziri A, et al. The effects of graft size and insertion site location during anterior cruciate ligament reconstruction on intercondylar notch impingement. Knee 2017;24:525-35. [Crossref] [PubMed]
- Mariscalco MW, Flanigan DC, Mitchell J, et al. The influence of hamstring autograft size on patient-reported outcomes and risk of revision after anterior cruciate ligament reconstruction: a Multicenter Orthopaedic Outcomes Network (MOON) Cohort Study. Arthroscopy 2013;29:1948-53. [Crossref] [PubMed]
- Morgan JA, Dahm D, Levy B, et al. Femoral tunnel malposition in ACL revision reconstruction. J Knee Surg 2012;25:361-8. [Crossref] [PubMed]
- Trojani C, Sbihi A, Djian P, et al. Causes for failure of ACL reconstruction and influence of meniscectomies after revision. Knee Surg Sports Traumatol Arthrosc 2011;19:196-201. [Crossref] [PubMed]
- Udagawa K, Niki Y, Enomoto H, et al. Factors influencing graft impingement on the wall of the intercondylar notch after anatomic double-bundle anterior cruciate ligament reconstruction. Am J Sports Med 2014;42:2219-25. [Crossref] [PubMed]
- Howell SM, Taylor MA. Failure of reconstruction of the anterior cruciate ligament due to impingement by the intercondylar roof. J Bone Joint Surg Am. 1993;75:1044-55. [Crossref] [PubMed]
- Burnham JM, Malempati CS, Carpiaux A, et al. Anatomic Femoral and Tibial Tunnel Placement During Anterior Cruciate Ligament Reconstruction: Anteromedial Portal All-Inside and Outside-In Techniques. Arthrosc Tech 2017;6:e275-82. [Crossref] [PubMed]
- Lee DH, Kim HJ, Ahn HS, et al. Comparison of Femoral Tunnel Length and Obliquity Between Transtibial, Anteromedial Portal, and Outside-In Surgical Techniques in Single-Bundle Anterior Cruciate Ligament Reconstruction: A Meta-analysis. Arthroscopy 2016;32:142-50. [Crossref] [PubMed]
- Herbort M, Domnick C, Raschke MJ, et al. Comparison of Knee Kinematics After Single-Bundle Anterior Cruciate Ligament Reconstruction via the Medial Portal Technique With a Central Femoral Tunnel and an Eccentric Femoral Tunnel and After Anatomic Double-Bundle Reconstruction: A Human Cadaveric Study. Am J Sports Med 2016;44:126-32. [Crossref] [PubMed]
- Osti M, Krawinkel A, Ostermann M, et al. Femoral and tibial graft tunnel parameters after transtibial, anteromedial portal, and outside-in single-bundle anterior cruciate ligament reconstruction. Am J Sports Med 2015;43:2250-8. [Crossref] [PubMed]
- Pascual-Garrido C, Swanson BL, Swanson KE. Transtibial versus low anteromedial portal drilling for anterior cruciate ligament reconstruction: a radiographic study of femoral tunnel position. Knee Surg Sports Traumatol Arthrosc 2013;21:846-50. [Crossref] [PubMed]
- Tompkins M, Milewski MD, Brockmeier SF, et al. Anatomic femoral tunnel drilling in anterior cruciate ligament reconstruction: use of an accessory medial portal versus traditional transtibial drilling. Am J Sports Med 2012;40:1313-21. [Crossref] [PubMed]
- Meyer GH. Die architektur der spongiosa. Arch Anat Physiol Wiss Med 1867;34:615-29.
- Frost HM. From Wolff's law to the Utah paradigm: insights about bone physiology and its clinical applications. Anat Rec 2001;262:398-419. [Crossref] [PubMed]
- Frost HM. Changing concepts in skeletal physiology: Wolff's Law, the Mechanostat, and the "Utah Paradigm". Am J Hum Biol 1998;10:599-605. [Crossref] [PubMed]
- McNeill Ingham SJ, de Carvalho RT, Abdalla RJ, et al. Bony Morphology: Comparative Anatomy and its Importance for the Anterior Cruciate Ligament. Oper Tech Orthop 2017;1:2-7. [Crossref]
- Dejour D, Saffarini M, Demey G, et al. Tibial slope correction combined with second revision ACL produces good knee stability and prevents graft rupture. Knee Surg Sports Traumatol Arthrosc 2015;23:2846-52. [Crossref] [PubMed]
Cite this article as: Burnham JM, Pfeiffer T, Shin JJ, Herbst E, Fu FH. Bony morphologic factors affecting injury risk, rotatory stability, outcomes, and re-tear rate after anterior cruciate ligament reconstruction. Ann Joint 2017;2:44.