Cruciate ligament-derived mesenchymal stem cells: a potential cell source for cartilage regeneration
Introduction
Tissue engineering based on stem cells is a promising strategy to repair the degenerate or damaged tissues (1-3). Mesenchymal stem cells (MSCs), unlike embryonic stem cells, are considered to be a potential cell source for tissue engineering for less controversy in ethical problems (4). The advantage of MSCs applied in tissue engineering is that these cells can differentiate into targeted tissues, including bone, adipose, cartilage, myoblasts, nucleus pulposus and so on (5-7). The self-renewal and proliferation abilities of MSCs are also robust with phenotype stability (8).
The prevalence of musculoskeletal diseases have motivated researchers to investigate the potential cell sources of MSCs derived from different tissues for tissue regeneration (9,10). Many tissue engineering researches have been based on the proliferation and differentiation properties of MSCs derived from multiple tissues (11). Bone marrow is a widely investigated source of MSCs, and bone marrow-derived mesenchymal stem cells (BMSCs) have been effectively used in tissue repair and regeneration (12,13). However, BMSCs can only be obtained through bone marrow biopsy, which will cause unnecessary pain. Adipose-derived mesenchymal stem cells (AMSCs) have attracted increasing interest due to easier isolation procedures and relative abundance in adipose tissues, representing a potential alternative to BMSCs (14,15). Recently, a population of multipotent MSCs was isolated from ligament tissues with similar properties of BMSCs (16,17).
Different sources of MSCs may exhibit different characteristics when applied in tissue regeneration (18). How the origin of bone marrow, adipose or cruciate ligament may affect MSCs’ ability to differentiate remains controversial and largely unknown. Our aim has been to compare the proliferation and induced differentiation potentials of MSCs isolated from bone marrow, adipose and cruciate ligament.
Methods
Cell isolation and culture
The Animal Research Ethics Committee, the Southeast University approved all experiments. Ten 6-week old male Sprague-Dawley rats weighing 200 to 220 g were used in this study. MSCs isolated were from the same animal. The procedures of the isolation of BMSCs and AMSCs have been previously established (19). Cruciate ligament-derived mesenchymal stem cells (CLMSCs) were isolated by explant culture system based on a study reported with some modifications (17). Briefly, the knee cruciate ligaments were carefully excised from healthy rats overdosed with 2.5% intraperitoneal sodium phenobarbital (1.0 mL/400 g). When opening the knee joint capsule, only the ligaments between the femoral condyle nest but not the meniscus tissues often free form the tibia were collected. The collected cruciate ligaments, including anterior and posterior cruciate ligaments were rinsed twice with sterile phosphate buffered saline (PBS). The possible blood vessels and sheath of the ligaments were carefully scraped and washed by PBS again. The washed tissues were minced into pieces of about 1 mm3 and placed in a 6-well plates at 37 °C for 5 minutes to promote adherence, followed by the addition of 2 mL basal complete culture medium consisting of DMEM supplemented with 10% fetal bovine serum (FBS) (Hyclone), antibiotics (50 IU penicillin/mL and 50 mg streptomycin/mL; Beijing Leagene Biotech, China) for 2 weeks. During this period, cells migrated from the tissue fragments, which formed a population of outgrowth cells. The isolated cells were sub-cultured when they reached 80% to 90% confluence. Medium was changed every 3 days. Third passage cells (P3) were used for all experiments.
Fluorescence-activated cell sorting analysis
BMSCs, AMSCs, and CLMSCs at P3 were utilized for surface marker expression by flow cytometry according to previously established procedures (20). The cultured cells were harvested and washed twice with cold PBS to remove the supernatants. Then the washed cells were resuspended and incubated for 30 min at 4 °C in the dark with phycoerythrin (PE) or fluorescein isothiocyanate (FITC)-conjugated monoclonal antibodies. Antibodies against CD29 and CD 45 were purchased from BD Biosciences. Antibodies against CD90 were purchased from Santa Cruz Biotech. Nonspecific IgG1 (PE) and IgG1 (FITC) (both from Santa Cruz Biotech) for all fluorochromes were used as comparative controls. Flow cytometry analysis was performed with a FACSAria (BD Biosciences).
Colony-forming assay
BMSCs, AMSCs and CLMSCs were plated at 300 cells per 10-cm2 dish, 10 dishes in each cell group. Each type of cells was cultured with complete medium for 14 days. Then, cells were washed 3 times by PBS, fixed with 10% methanol for 15 min and stained with 0.5% crystal violet (Sigma, St. Louis, MO, USA) for 15 min to count the number of cell colonies. Colonies smaller than 2 mm in diameter were ignored. The number of colonies in each plate was reported.
Cell proliferation assay
BMSCs, AMSCs and CLMSCs were plated at 5,000 cells/well in a 96-well plate and incubated at 37 °C, 5% CO2 for 2 days. Cell proliferation was assessed using the Cell Counting Kit-8 (Qihai-futai Bio Tec, Shanghai, China) according to the manufacturer’s instruction. The absorbance value was measured at 370 to 450 nm.
Expression of osteogenic, adipogenic, and chondrogenic markers at basal state using quantitative real-time polymerase chain reaction (qRT-PCR)
The expression of osteogenic, chondrogenic, and adipogenic markers at basal state was measured using qRT-PCR as previously described (21). Briefly, BMSCs, AMSCs and CLMSCs at P3 were harvested and homogenized for RNA extraction using Trizol (Invitrogen, USA) in triplicate. The mRNA was reverse transcribed to complementary DNA (cDNA) using the Omniscript RT kit (Qiagen, USA). mRNA levels were measured by qRT-PCR (Stepone real-time PCR Applied Biosystems, USA) using the Fast EvaGreen® master mix for quantitative and high-resolution melting PCR (Biotium, USA). The 20-μL reaction contained 1 μL cDNA from each sample mixed with 10 μL 2X Fast EvaGreen® qPCR Master Mix, 2 μL 10X ROX of the assays-on-demand kit (Applied Biosystems), 1 μL primer, and 6 μL RNase/DNase-free water. The PCR conditions were: incubation at 95 °C for 10 min followed by 45 cycles at 95 °C for 5 s and at 60 °C for 1 min. Data was analyzed using the ABI Stepone Sequence Detection Systems software, version 1.0, supplied by Applied Biosystems. The expression of target gene was normalized to that of GAPDH. Relative gene expression was calculated using formula 2−ΔCt. Specific primers for adipogenic markers [peroxisome proliferator-activated receptor gamma 2 (PPARγ2) and CCAAT enhancer binding protein alpha (c/EBPα)], osteogenic markers [Alpl, osteocalcin (Bglap), Runx2, bone morphogenetic protein 2 (Bmp2) and osteopontin (Spp1)], and chondrogenic markers (Col2A1, Acan, and Sox9) were listed in Table 1.
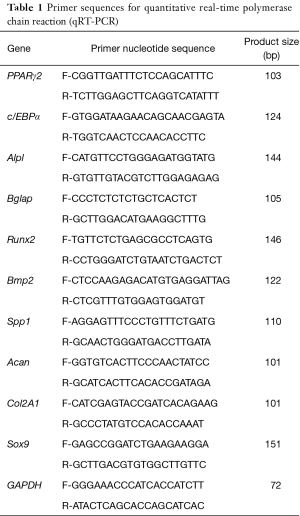
Full table
Adipogenic differentiation assay
BMSCs, AMSCs and CLMSCs were plated at 5×103 cells/cm2 in a 6-well plate and cultured with complete medium until the cells reached 90–100% confluence. The complete medium was then still cultured with complete medium or adipogenic medium (RASMX-90031, Cyagen Biosciences Inc., Goleta, CA). The medium was replaced every 3 days. At days 21, the oil droplets were assessed using an Oil red O staining assay. Briefly, the cells were washed twice in PBS, fixed in 10% formaldehyde for 10 min at room temperature, and stained with 0.3% filtered Oil red O staining solution (sigma) for 2 hours. The mRNA expression of adipogenic markers PPARγ2 and c/EBPα were assessed at days 21 by qRT-PCR using primers listed in Table 1.
Osteogenic differentiation assay
BMSCs, AMSCs and CLMSCs were plated at 4×103 cells/cm2 in a 6-well plate and cultured in basal complete culture medium until the cells reached confluence. They were then incubated in basal complete medium or osteogenic medium (RASMX-90021, Cyagen Biosciences Inc., Goleta, CA, USA). At days 21, the calcium nodule formation was assessed using an Alizarin red staining assay. Briefly, the cell layer was washed with PBS twice, fixed with 10% ethanol, and then washed with pure water twice. For Alizarin red staining, the cells were stained with 0.5% Alizarin red S (pH 4.1, sigma) for 10 min, and washed with pure water twice and then air-dried. The mRNA expression of osteogenic markers Alpl, Bglap, Runx2, Bmp2, Spp1 were assessed at days 21 by qRT-PCR using primers listed in Table 1.
Chondrogenic differentiation assay
Chondrogenic differentiation was assessed by the pellet culture method as modified recently. A total of 5×105 cells were centrifugated at 450 g for 10 min to form a micromass in a 15-mL conical polypropylene tube and cultured in complete or chondrogenic medium (RASMX-90041, Cyagen Biosciences Inc., Goleta, CA, USA) at 37 °C 5% CO2 (R&D Systems, Minneapolis, MN, USA). At days 21, the pellet was fixed for and toluidine blue staining for the examination of glycosaminoglycan deposition. The mRNA expression of Col2A1, Acan, and Sox9 were studied at days 21 by qRT-PCR using primers listed in Table 1.
Statistic analysis
Data was presented as mean ± SD and determined by one-way analysis of variance (ANOVA). If there was a significant overall difference between groups, pairwise comparisons were conducted using Scheffe’s post hoc test. All data analysis was done using IBM Statistical Package for the Social Sciences, version 19.0 (SPSS Inc.). Values of P<0.05 were considered statistically significant.
Results
Cell morphology
BMSCs showed a homogeneous morphology (Figure 1A), whereas AMSCs were heterogeneous varying from elongated cells to flat round cells at P0 (Figure 1B). CLMSCs migrated from the cut ends of tissue explants after a lag of 5 days’ culture. At P0, CLMSCs were spindle and fiber-like (Figure 1C). At P3, BMSCs and AMSCs demonstrated similar spindle-shaped and fibroblast-like morphology (Figure 1D,E). At P3, CLMSCs also exhibited fibroblast-like morphology like BMSCs and AMSCs (Figure 1F).
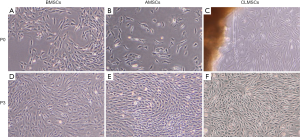
Fluorescence-activated cell sorting analysis
Flow cytometry analysis (Figure 2) demonstrated that BMSCs, AMSCs and CLMSC s were all positive for CD29 and CD90, while showed negligible expression of CD45.
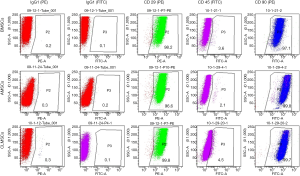
Clonogenicity and proliferative potential
AMSCs and CLMSCs formed more colonies (P<0.05) (Figure 3A,B) and proliferated faster (P<0.05) (Figure 3C) than BMSCs. CLMSCs exhibited superior colony forming and proliferation potentials. However, the colony numbers and absorbance value between CLMSCs and AMSCs were not of statistical significance (P<0.05) (Figure 3B,C).
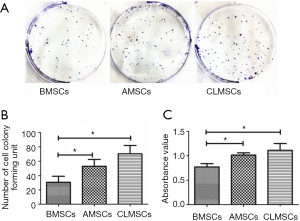
Expression of adipogenic, osteogenic and chondrogenic markers at basal state
AMSCs showed the highest mRNA level of adipogenic (PPARγ2 and c/EBPα) markers among these three types of MSCs in basal complete culture medium (P<0.05) (Figure 4A,B). BMSCs expressed the highest mRNA level of osteogenic (Alpl, Bglap, Runx2, Bmp2, Spp1) markers in basal complete culture medium (P<0.05) (Figure 4C-G). CLMSCs exhibited the highest mRNA level of chondrogenic (Col2A1, Acan, and Sox9) markers in basal complete culture medium (P<0.05) (Figure 4H-J).
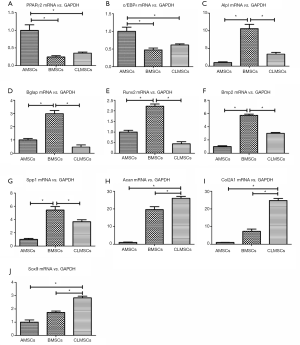
Adipogenic differentiation potential
Indistinguishable positive Oil droplets were formed in BMSCs, AMSCs and CLMSCs upon adipogenic induction for 21 days (Figure 5A-C). Under the same induction conditions, the expression of adipogenic markers, including PPARγ2 and c/EBPα, was significantly higher in AMSCs than those in BMSCs and CLMSCs (P<0.05, Figure 5D,E).
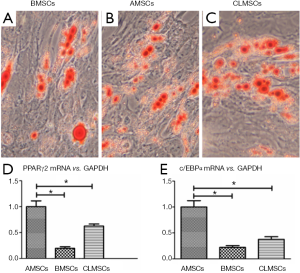
Osteogenic differentiation potential
There was significant increase in matrix mineralization in BMSCs, AMSCs and CLMSCs upon osteogenic induction for 21 days, as demonstrated by positive Alizarin red staining (Figure 6A-C). Under the same induction conditions, the expression of osteogenic markers, including Alpl, Bglap, Runx2, Bmp2, Spp1, was significantly higher in BMSCs than those in AMSCs and CLMSCs (P<0.05, Figure 6D-H).
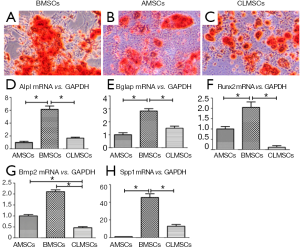
Chondrogenic differentiation potential
Cell pellets were formed at days 21 upon chondrogenic induction in all cell types. The cartilaginous phenotype of all induced cells was confirmed by positive toluidine blue staining in BMSCs, AMSCs and CLMSCs (Figure 7A-C). Magnification: ×200. Under the same induction condition, the expression of chondrogenic markers, including Col2A1, Acan and Sox9, was significantly higher in CLMSCs than those in BMSCs and AMSCs (P<0.05, Figure 7D-F).
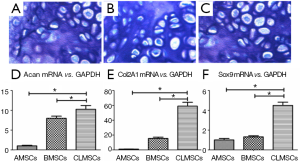
Discussion
MSCs have been widely investigated and studied for their regenerative capacity. Previous pre-clinical studies have indicated that MSCs isolated from different tissues, including bone marrow, adipose, cruciate ligament and cord blood, can be applied in tissue repair, exhibiting promising therapeutic potentials (22-24). How to choose an appropriate cell source is an important issue when designing an optimal cell therapy, because MSCs isolated from different tissues may have specific proliferation and differentiation properties (25). This study therefore aimed to compare the colony-forming ability, proliferative potential, and multilineage differentiation potentials of BMSCs, AMSCs and CLMSCs.
Microscopic observation during expansion has shown that BMSCs, AMSCs and CLMSCs displayed similar morphology after three passages, which was plastic-adherent with a fibroblast-like spindle-shaped morphology. CLMSCs is a recently isolated MSCs with robust proliferation and differentiation potential (17). According to our best knowledge, the proliferation potential of CLMSCs was not simultaneously analyzed when compared to BMSCs and AMSCs previously. This study suggested that CLMSCs and AMSCs showed superior colony forming and proliferation capacity when compared to BMSCs. In a comparative study of CLMSCs and BMSCs, CLMSCs proliferated faster than BMSCs, which bore a resemblance with our study (26).
MSCs are pluripotent cells identified primarily in bone marrow. Similar cells were reported in multiple tissues, including fat (27), muscle (28), tendon (29), synovium (9) and so on. According to the phenotypic analysis reported, BMSCs and AMSCs are positive for stromal-cell-associated markers, such as CD73, CD29, and CD90 while negative for endothelial markers, including CD45, CD14, CD34 and CD31. CD105, CD13 and CD44 are also expressed by both cell types. Our results showed that BMSCs and AMSCs isolated were positive for CD29, CD90, while negative for CD45, which was consistent with previous studies reported (30,31).
We further showed the highest adipogenic markers in AMSCs, osteogenic markers in BMSCs and chondrogenic markers in CLMSCs at basal state. Our results suggest that AMSCs are more promising cell source for adipose-related tissue regeneration while BMSCs are superior in bone regeneration. This result corroberated with the findings of previous studies (32-34). Interestingly, we noticed that it was CLMSCs, but not BMSCs that exhibited superior chondrogenesis potential. To our best knowledge, it is the first time that BMSCs and CLMSCs were compared at basal state for chondrogenesis-related mRNA expression. Because of higher chondrogenic-relate mRNA expression and proliferation potential, CLMSCs might be a competitive cell source for cartilage regeneration.
When designing a tissue engineered therapy, an in vitro pre-induction process for MSCs is often necessary. To confirm the in vitro differentiation property of BMSCs, AMSCs and CLMSCs, we induced these three types of MSCs towards adipogenic, osteogenic and chondrogenic lineages by the same induction agents. Our results showed that after 21 days induction, adipogenic differentiation in BMSCs, AMSCs and CLMSCs were demonstrated by the well-acknowledged Oil red staining. The stainings among these three types of MSCs are indistinguishable and strongly positive. mRNA analysis revealed that under the same induction conditions, AMSCs expressed the highest level of adipogenic markers, including PPARγ2 and c/EBPα. Similar positive Alizarin red staining and toluidine blue staining were observed in each cell group after 21 days induction. However, BMSCs showed the highest osteogenic-related mRNA while CLMSCs expressed the highest chondrogenic-related mRNA. The results are similar to a study of Cheng et al. (26), who demonstrated that BMSCs exhibited a higher osteogenic capacity and similar chondrogenic potential compared to CLMSCs. However, studies of different results also exist. Steinert et al. (17) reported that BMSCs had a higher chondrogenic potential and similar osteogenic potential compared to CLMSCs. Our investigations provided insights into the cellular characteristics of BMSCs, AMSCs and CLMSCs. However, the present study is limited to the in vitro nature. The in vitro higher mRNA expression does not necessary guarantee the advantages of the in vivo regenerative effect, because gene transfection, cell scaffold and growth factors when co-applied together can also affect the differentiation process of transplanted MSCs. Thus, further in vivo studies are required to clarify their relevance to tissue regeneration of different properties.
The present study compared the biological characteristics of BMSCs, AMSCs and CLMSCs. Our study showed that, despite many similarities among these three types of MSCs, there are differences existing in proliferation and induced differentiation properties, which can offer insights in choosing the most appropriate cell source for tissue engineering strategies. CLMSCs and AMSCs showed superiority in cell proliferation compared to BMSCs. BMSCs is more promising for bone regeneration; AMSCs is superior in adipose tissue applications; CLMSCs might offer a potential cell source in cartilage regeneration.
Acknowledgments
Funding: This work was supported by Science and Technology Bureau of Nanjing, Jiangsu, China (Grant No. 7990000031).
Footnote
Conflicts of Interest: All authors have completed the ICMJE uniform disclosure form (available at http://dx.doi.org/10.21037/aoj.2016.05.01). The authors have no conflicts of interest to declare.
Ethical Statement: The authors are accountable for all aspects of the work in ensuring that questions related to the accuracy or integrity of any part of the work are appropriately investigated and resolved. The Animal Research Ethics Committee, the Southeast University approved all experiments.
Open Access Statement: This is an Open Access article distributed in accordance with the Creative Commons Attribution-NonCommercial-NoDerivs 4.0 International License (CC BY-NC-ND 4.0), which permits the non-commercial replication and distribution of the article with the strict proviso that no changes or edits are made and the original work is properly cited (including links to both the formal publication through the relevant DOI and the license). See: https://creativecommons.org/licenses/by-nc-nd/4.0/.
References
- Polak JM, Bishop AE. Stem cells and tissue engineering: past, present, and future. Ann N Y Acad Sci 2006;1068:352-66. [Crossref] [PubMed]
- Gomillion CT, Burg KJ. Stem cells and adipose tissue engineering. Biomaterials 2006;27:6052-63. [Crossref] [PubMed]
- Howard D, Buttery LD, Shakesheff KM, et al. Tissue engineering: strategies, stem cells and scaffolds. J Anat 2008;213:66-72. [Crossref] [PubMed]
- Liao HT, Chen CT. Osteogenic potential: Comparison between bone marrow and adipose-derived mesenchymal stem cells. World J Stem Cells 2014;6:288-95. [Crossref] [PubMed]
- Chamberlain G, Fox J, Ashton B, et al. Concise review: mesenchymal stem cells: their phenotype, differentiation capacity, immunological features, and potential for homing. Stem Cells 2007;25:2739-49. [Crossref] [PubMed]
- Fu Y, Li R, Zhong J, et al. Adipogenic differentiation potential of adipose-derived mesenchymal stem cells from ovariectomized mice. Cell Prolif 2014;47:604-14. [Crossref] [PubMed]
- Feng G, Jin X, Hu J, et al. Effects of hypoxias and scaffold architecture on rabbit mesenchymal stem cell differentiation towards a nucleus pulposus-like phenotype. Biomaterials 2011;32:8182-9. [Crossref] [PubMed]
- Phinney DG, Prockop DJ. Concise review: mesenchymal stem/multipotent stromal cells: the state of transdifferentiation and modes of tissue repair--current views. Stem Cells 2007;25:2896-902. [Crossref] [PubMed]
- Sakaguchi Y, Sekiya I, Yagishita K, et al. Comparison of human stem cells derived from various mesenchymal tissues: superiority of synovium as a cell source. Arthritis Rheum 2005;52:2521-9. [Crossref] [PubMed]
- Wang SZ, Rui YF, Lu J, et al. Cell and molecular biology of intervertebral disc degeneration: current understanding and implications for potential therapeutic strategies. Cell Prolif 2014;47:381-90. [Crossref] [PubMed]
- Tuan RS, Boland G, Tuli R. Adult mesenchymal stem cells and cell-based tissue engineering. Arthritis Res Ther 2003;5:32-45. [Crossref] [PubMed]
- Kagami H, Agata H, Tojo A. Bone marrow stromal cells (bone marrow-derived multipotent mesenchymal stromal cells) for bone tissue engineering: basic science to clinical translation. Int J Biochem Cell Biol 2011;43:286-9. [Crossref] [PubMed]
- Grayson WL, Bunnell BA, Martin E, et al. Stromal cells and stem cells in clinical bone regeneration. Nat Rev Endocrinol 2015;11:140-50. [Crossref] [PubMed]
- Strioga M, Viswanathan S, Darinskas A, et al. Same or not the same? Comparison of adipose tissue-derived versus bone marrow-derived mesenchymal stem and stromal cells. Stem Cells Dev 2012;21:2724-52. [Crossref] [PubMed]
- Zhu X, Shi W, Tai W, et al. The comparition of biological characteristics and multilineage differentiation of bone marrow and adipose derived Mesenchymal stem cells. Cell Tissue Res 2012;350:277-87. [Crossref] [PubMed]
- Cheng MT, Yang HW, Chen TH, et al. Isolation and characterization of multipotent stem cells from human cruciate ligaments. Cell Prolif 2009;42:448-60. [Crossref] [PubMed]
- Steinert AF, Kunz M, Prager P, et al. Mesenchymal stem cell characteristics of human anterior cruciate ligament outgrowth cells. Tissue Eng Part A 2011;17:1375-88. [Crossref] [PubMed]
- Richardson SM, Hoyland JA, Mobasheri R, et al. Mesenchymal stem cells in regenerative medicine: opportunities and challenges for articular cartilage and intervertebral disc tissue engineering. J Cell Physiol 2010;222:23-32. [Crossref] [PubMed]
- Uysal CA, Tobita M, Hyakusoku H, et al. The Effect of Bone-Marrow-Derived Stem Cells and Adipose-Derived Stem Cells on Wound Contraction and Epithelization. Adv Wound Care (New Rochelle) 2014;3:405-413. [Crossref] [PubMed]
- Liu LT, Huang B, Li CQ, et al. Characteristics of stem cells derived from the degenerated human intervertebral disc cartilage endplate. PLoS One 2011;6:e26285 [Crossref] [PubMed]
- Wang SZ, Chang Q, Kong XF, et al. The chondrogenic induction potential for bone marrow-derived stem cells between autologous platelet-rich plasma and common chondrogenic induction agents: a preliminary comparative study. Stem Cells Int 2015;2015:589124.
- Parekkadan B, Milwid JM. Mesenchymal stem cells as therapeutics. Annu Rev Biomed Eng 2010;12:87-117. [Crossref] [PubMed]
- Vishnubalaji R, Al-Nbaheen M, Kadalmani B, et al. Comparative investigation of the differentiation capability of bone-marrow- and adipose-derived mesenchymal stem cells by qualitative and quantitative analysis. Cell Tissue Res 2012;347:419-27. [Crossref] [PubMed]
- Beane OS, Fonseca VC, Cooper LL, et al. Impact of aging on the regenerative properties of bone marrow-, muscle-, and adipose-derived mesenchymal stem/stromal cells. PLoS One 2014;9:e115963 [Crossref] [PubMed]
- Satija NK, Singh VK, Verma YK, et al. Mesenchymal stem cell-based therapy: a new paradigm in regenerative medicine. J Cell Mol Med 2009;13:4385-402. [Crossref] [PubMed]
- Cheng MT, Liu CL, Chen TH, et al. Comparison of potentials between stem cells isolated from human anterior cruciate ligament and bone marrow for ligament tissue engineering. Tissue Eng Part A 2010;16:2237-53. [Crossref] [PubMed]
- Knippenberg M, Helder MN, Zandieh Doulabi B, et al. Osteogenesis versus chondrogenesis by BMP-2 and BMP-7 in adipose stem cells. Biochem Biophys Res Commun 2006;342:902-8. [Crossref] [PubMed]
- Qu-Petersen Z, Deasy B, Jankowski R, et al. Identification of a novel population of muscle stem cells in mice: potential for muscle regeneration. J Cell Biol 2002;157:851-64. [Crossref] [PubMed]
- Zhang J, Wang JH. Characterization of differential properties of rabbit tendon stem cells and tenocytes. BMC Musculoskelet Disord 2010;11:10. [Crossref] [PubMed]
- Sousa BR, Parreira RC, Fonseca EA, et al. Human adult stem cells from diverse origins: an overview from multiparametric immunophenotyping to clinical applications. Cytometry A 2014;85:43-77. [Crossref] [PubMed]
- Lin CS, Xin ZC, Dai J, et al. Commonly used mesenchymal stem cell markers and tracking labels: Limitations and challenges. Histol Histopathol 2013;28:1109-16. [PubMed]
- Niemeyer P, Fechner K, Milz S, et al. Comparison of mesenchymal stem cells from bone marrow and adipose tissue for bone regeneration in a critical size defect of the sheep tibia and the influence of platelet-rich plasma. Biomaterials 2010;31:3572-9. [Crossref] [PubMed]
- Zhang W, Zhang X, Wang S, et al. Comparison of the use of adipose tissue-derived and bone marrow-derived stem cells for rapid bone regeneration. J Dent Res 2013;92:1136-41. [Crossref] [PubMed]
- Hayashi O, Katsube Y, Hirose M, et al. Comparison of osteogenic ability of rat mesenchymal stem cells from bone marrow, periosteum, and adipose tissue. Calcif Tissue Int 2008;82:238-47. [Crossref] [PubMed]
Cite this article as: Wang SZ, Hao ZC, Ji ML, Zhang XJ, Lu J. Cruciate ligament-derived mesenchymal stem cells: a potential cell source for cartilage regeneration. Ann Joint 2016;1:6.