Clinical anatomy and stabilizers of the glenohumeral joint
Introduction
Shoulder motion requires the coordinated effort of muscles, tendons, ligaments, and bones primarily across the glenohumeral joint and scapulothoracic articulation (1). The glenohumeral joint is a multiaxial synovial ball and socket joint that functions as a diarthrosis to facilitate a wide range of motion for the upper extremity (2). Stability across the glenohumeral joint is balanced by both static and dynamic mechanisms (1). Lifetime prevalence rates of shoulder pain in the general population range from 6.7% to 66.7% (3). Shoulder instability among young, active patients is a common clinical problem with reported incidence ranging from 0.08 to 0.24 per 1,000 person-years in the United States and Europe, respectively (4,5). While functional rehabilitation and surgical management aim to improve glenohumeral pain, function and stability, approximately 70% of patients fail to regain normal joint function despite treatment (6). Given the prevalence of glenohumeral pathology and significant rehabilitation potential, a comprehensive understanding of the structure and function of this joint is germane to the practicing sports medicine specialist.
General anatomy
The glenohumeral joint consists of an articulation between the scapula and humerus (Figure 1). The humeral head lies within the glenoid fossa, a cavity that is lined by the glenoid labrum. The shallow nature of the glenoid fossa lends the glenohumeral joint an increased range of motion while providing little stability. The osseous structures are surrounded by the glenohumeral capsule, a fibrous network attached medially to the margin of the glenoid cavity and laterally to the anatomic neck of the humerus (7,8). Thickenings within the glenohumeral capsule comprise the superior, middle, and inferior glenohumeral ligaments (IGHLs), which are critical static stabilizers of the joint (8). The rotator cuff muscles surround the glenohumeral capsule and create balanced force couples that play a central role as dynamic stabilizers of the humeral head during range of motion.
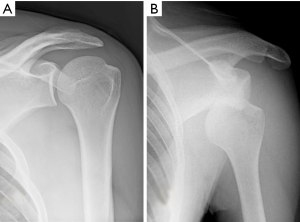
Planes of motion
Glenohumeral motion primarily occurs in the axial, sagittal, coronal, and scapular planes. Flexion and extension of the upper extremity occurs in the sagittal plane, internal and external rotation in the axial plane, and abduction and adduction in the coronal plane. The scapular plane is defined by an angle made between the spine of the scapula and coronal plane and is positioned approximately at a right angle to the plane of the glenoid (7,9). The scapular plane ranges from 30°–45° anterior to the coronal plane. Abduction in the coronal plane requires external rotation of the humerus to avoid impingement of the greater tuberosity of the humerus on the acromion. This is not required with movement in the scapular plane (9). Movement throughout these planes requires coordination of many muscles, mainly the rotator cuff and deltoid muscles, along with the biceps brachii, latissimus dorsi, pectoralis major, teres minor, and scapulothoracic musculature (9).
The deltoid muscle is multipennate and comprised of anterior fibers, which originate from the clavicle; middle fibers, which arise from the lateral acromion; and posterior fibers, which attach to the spine of the scapula. The axillary nerve innervates these fibers and powers forward flexion, abduction, and extension of the arm, respectively, and collectively insert on the deltoid tuberosity on the lateral margin of the humeral shaft (10). The deltoid is the prime mover during shoulder abduction and is more effective at higher abduction angles (11,12). Due to the vector created by the deltoid’s muscle fibers, optimal abduction force is generated with motion in the scapular plane (9). With shoulder impingement, a greater degree of deltoid recruitment occurs at decreased angles of abduction (0°–30°) to compensate for decreased rotator cuff activation (11).
Static stabilizers
Osteology
The humeral head is spheroidal in shape in approximately 90% of individuals with an average diameter of 43 millimeters (13). The main blood supply to the humeral head is the posterior humeral circumflex artery (14). Hyaline articular cartilage lines the proximal end from the level of the anatomic neck. Cartilage depth is thickest at the axial center of the humeral head and thins peripherally (15). The articular surface is inclined upward on average 130 degrees with an average retroversion angle of 30 degrees; both measures, however, are highly variable (16). The retroversion angle of the humeral head is defined by the orientation of the articular surface with respect to the transepicondylar axis of the distal humerus (16). Lateral to the humeral head is the greater tuberosity, which serves as the insertion site for the supraspinatus, infraspinatus and teres minor. Distal to the anatomic neck is the lesser tuberosity, the insertion site of the subscapularis. Between the tuberosities is the bicipital groove, a critical landmark during surgical fracture repair and prosthesis alignment during shoulder arthroplasty (17,18). One morphometric study showed the average groove length to be 8.1 cm with a depth of 4.0 mm (19).
The glenoid is an inverted pear-shaped concave surface that articulates with 33% of the humeral head in formation of the glenohumeral joint which supports humeral head rotation and gliding (20). This lateral scapular projection is retroverted on average 5 degrees from the scapular body (range, 7 degrees retroversion to 10 degrees anteversion) and tilted superiorly on average 5 degrees with respect to the floor of the supraspinatus fossa (20). Glenoid cartilage is thicker at its periphery and thinner at its axial center and may have a bare area at this location (15). The area of highest contact pressure during normal glenohumeral motion is located posterosuperior on the glenoid (21). Synovial fluid within the joint permits an adhesion-cohesion relationship or “suction-cup effect”.
Labral and capsular anatomy
The glenoid labrum is a fibrocartilaginous rim of tissue that is triangular in cross-section and acts to increase the depth of the glenoid vault (Figure 2). It serves as a passive stabilizer of the joint while providing an insertion for the anterior band of the IGHL and long head of the biceps (22). Anatomic variants of the labrum, particularly in the anterosuperior quadrant have been well described in the literature (23). Anterosuperior labral tissue anchored to the glenoid rim in association with a flat or broad middle glenohumeral ligament (MGHL) is the most common “normal” variant. Less common variants include a sublabral foramen and Buford complex (23). The Buford complex is defined by a cord-like MGHL with attachment to the base of the biceps anchor and complete absence of the anterosuperior labrum. Intraoperative identification of this anatomic variant is critical, as arthroscopic repair of a Buford complex will lead to painful and restricted external rotation when the arm is in an adducted position.
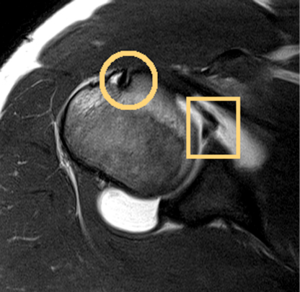
The glenohumeral capsule functions in multiple planes to provide static stability to the glenohumeral joint and maintain its synovial environment (24). Classic teaching describes discrete capsular thickenings defined as the superior glenohumeral ligament, MGHL, and IGHL complex. The superior glenohumeral ligament is least variable among the capsular ligaments and becomes taut with the arm in adduction to act as a restraint to inferior translation (25). The MGHL is the most variable capsular ligament and functions primarily to prevent anterior-posterior translation in the mid-range of abduction. The IGHL complex is most commonly injured and has been described as having three distinct anatomic regions: superior band, anterior axillary pouch, and posterior axillary pouch (26). The anterior band anchors to the anterior labrum and serves as the primary restraint to anterior/inferior translation in the abducted/externally rotated late cocking position. This may serve as the weak link that predisposes patients to Bankart lesions with traumatic anterior shoulder instability. Experimental and computational studies have isolated these discrete ligamentous structures but more recent data suggests that these regions function in concert as a continuous sheet of fibrous tissue (27-29).
Dynamic stabilizers
Rotator cuff
The rotator cuff is defined as a collective group of four muscles: subscapularis, supraspinatus, infraspinatus, and teres minor. During abduction, the rotator cuff depresses the humeral head into the glenoid to allow the deltoid muscle to elevate the arm (30). In a normal shoulder, the rotator cuff also acts to stabilize anterior-posterior translation of the humeral head via compression to the glenoid by the combined antagonistic forces of the subscapularis (anterior) and the infraspinatus/teres minor (posterior).
The supraspinatus originates in the supraspinous fossa of the scapula and inserts at the superior facet of the greater tuberosity on the humerus (8). The suprascapular nerve that arises from the upper trunk of the brachial plexus innervates the muscle. The footprint of the supraspinatus has been described as triangular in shape with an average maximum medial-to-lateral length of 6.9 mm and anterior-to-posterior width of 12.6 mm (31). The supraspinatus abducts and to a lesser extent, externally rotates the humerus while exerting its greatest force at lower abduction angles, peaking between 0°–15° (12). Computer simulations have noted the contribution of the supraspinatus to resist inferior gravitational translation of the humeral head generated by the body weight of the arm (32).
The infraspinatus is a triangular shaped muscle that originates from the infraspinous fossa of the scapula and inserts on the middle facet of the greater tuberosity. The suprascapular nerve traverses through the spinoglenoid notch to innerve the infraspinatus. The teres minor originates at the dorsal surface of the inferior angle of the scapula and inserts over the medial lip of the intertubercular groove of the humerus (8). Together, these muscles comprise the posterior rotator cuff, acting to compress the glenohumeral joint, externally rotate and abduct the humerus, and counter anterior and superior translation of the humeral head within the glenohumeral joint (12). During overhead movement, these muscles provide external rotation that clears the greater tuberosity from the coracoacromial arch, effectively decreasing subacromial impingement (12). The infraspinatus is most effective at lower abduction angles, with the optimal isolation angle for this muscle occurring at 0° abduction (12). Infraspinatus force generation during abduction is greatest when the arm is held in internal rotation (7). The teres minor, however, has little effect on abduction due to its lower insertion on the scapula and humerus but does exert a consistent external rotation force over a wide range of abduction angles (12).
The subscapularis originates from the subscapular fossa, passes inferior to the coracoid, and becomes tendinous at the level of the glenoid before inserting at the lesser tuberosity and into the anterior glenohumeral capsule (Figure 3). This is the largest and strongest rotator cuff muscle. The upper and lower subscapular nerves from the posterior cord of the brachial plexus provide its innervation. The muscle functions to stabilize the glenohumeral joint anteriorly and facilitates internal rotation and abduction of the humerus (8,9). Peak internal rotation torque is generated at 0o abduction whereas abduction forces are greatest as abduction and external rotation angles increased (12). A cadaveric study found glenohumeral instability superiorly when the subscapularis, infraspinatus, and teres minor were paralyzed, highlighting the coordinated role of these force couples (33).
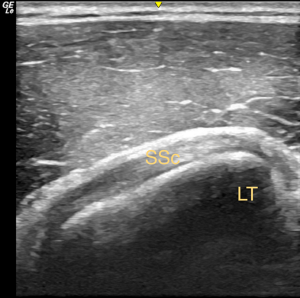
Biceps brachii
The biceps brachii muscle is bipennate, with a short head originating from the tip of the coracoid process of the scapula and a long head at the supraglenoid tubercle of the scapula with variable origin to the glenoid labrum. The origin of the long head has been shown to be highly variable (34). Four origin types with reference to 12-o’clock on the glenoid face have been described: entirely posterior, posterior dominant (most common), equal, and entirely anterior (34). Both muscle bellies give rise to a thick tendon that inserts distally at the radial tuberosity and a broad aponeurosis (lacertus fibrosus) that reinforces the cubital fossa before inserting onto the deep fascia of the forearm (8).
The biceps brachii is the main forearm supinator but also contributes to elbow flexion in addition to a secondary role in glenohumeral joint stability. Loading of the long head of the biceps brachii limits anterior translation and torsional forces of the humeral head as well as stress on the IGHL (35). These effects serve to center the humeral head within the glenohumeral joint and act as a fulcrum for arm elevation (35).
Periscapular muscles
Periscapular muscles serve to anchor the scapula allowing it to function as the base of the glenohumeral joint. This muscle group includes the pectoralis minor, serratus anterior, rhomboids, latissimus dorsi, and trapezius. Activation of these muscles during glenohumeral motion contributes to overall stability (36).
The pectoralis minor originates at the third through fifth ribs near the costal cartilage and inserts at the medial border and superior surface of the coracoid process of the scapula (36,37). It acts during scapular downward rotation, internal rotation, and anterior tilt (11). Contraction of the pectoralis minor decreases the subacromial space through anterior tilting and internal rotation of the scapula, serving as a mechanism of impingement (37). The serratus anterior originates at the external surfaces of the lateral portions of the first through eighth ribs and inserts over the anterior surface of the medial border of the scapula (36). The serratus anterior is typically divided into upper, middle, and lower portions. The upper portion has been minimally investigated. In studying the middle and lower serratus anterior, their positions provide an advantage for scapular upward rotation, posterior tilt, and external rotation (9,11). Due to their ability to approximate the scapula to the thorax during arm elevation, the middle and lower serratus anterior muscles play a pivotal role in reducing the risk of shoulder impingement symptoms (11). In addition, paralysis of the serratus anterior through disruption of the long thoracic nerve, results in a ‘winged scapula’ with the medial border and inferior angle of the scapula dissociating from the posterior thoracic wall (36).
The rhomboid major originates at the thoracic spinous processes of T2 through T5 and the rhomboid minor originates in the lower cervical spine from C7 through T1. Together they insert at the medial border of the scapula from the level of the spine to the inferior angle (8). The rhomboids are one of the primary movers in scapular retraction and downward rotation (9,11). The rhomboids act synergistically with the levator scapulae to resist lateral translation forces exerted by the serratus anterior (11). The latissimus dorsi originates at the spinous processes of T7 through T12, thoracolumbar fascia, iliac crest, and inferior third or fourth ribs. It inserts over the floor of the intertubercular groove of the humerus acts as an adductor and extensor of the glenohumeral joint, serving as an antagonist to the deltoid (1,8,36).
The trapezius is the largest muscle of this group. It originates from the occiput, nuchal ligament, and spinous processes of C7 through T12. The upper portion of the trapezius inserts at the distal third of the clavicle and acromion, the middle and lower portions of the trapezius insert across the scapular spine from the acromial process to its root (11,36). With regard to humeral movements, the trapezius functions primarily during abduction, with little contribution to flexion (11). The upper trapezius is most active during scapular elevation, functioning through elevation and retraction of the clavicle at the sternoclavicular joint. The middle and lower portions are positioned for scapular stabilization and external rotation (11). Paralysis of the trapezius muscle leads to downward rotation and lateral translation of the scapula (11). Paralysis of the trapezius muscle decreases stability of the shoulder girdle, and renders other stabilizing muscles, particularly the rhomboids and levator scapulae ineffective (11).
Conclusions
The shoulder is a complex unit of osseous, capsuloligamentous, and musculotendinous structures that maintain stability while permitting a wide range of motion for upper extremity function. Anatomic variability is common and as such, the treating physician should be well advised to have a comprehensive understanding of normal glenohumeral anatomy in order to restore appropriate function in pathologic conditions.
Acknowledgments
Funding: None.
Footnote
Provenance and Peer Review: This article was commissioned by the Guest Editors (Albert Lin and Jason J. Shin) for the series “Trends in Anterior Shoulder Instability” published in Annals of Joint. The article has undergone external peer review.
Conflicts of Interest: All authors have completed the ICMJE uniform disclosure form (available at http://dx.doi.org/10.21037/aoj.2017.10.03). The series “Trends in Anterior Shoulder Instability” was commissioned by the editorial office without any funding or sponsorship. The authors have no other conflicts of interest to declare.
Ethical Statement: The authors are accountable for all aspects of the work in ensuring that questions related to the accuracy or integrity of any part of the work are appropriately investigated and resolved.
Open Access Statement: This is an Open Access article distributed in accordance with the Creative Commons Attribution-NonCommercial-NoDerivs 4.0 International License (CC BY-NC-ND 4.0), which permits the non-commercial replication and distribution of the article with the strict proviso that no changes or edits are made and the original work is properly cited (including links to both the formal publication through the relevant DOI and the license). See: https://creativecommons.org/licenses/by-nc-nd/4.0/.
References
- Hawkes DH, Alizadehkhaiyat O, Fisher AC, et al. Normal shoulder muscular activation and co-ordination during a shoulder elevation task based on activities of daily living: An electromyographic study. J Orthop Res 2012;30:53-60. [Crossref] [PubMed]
- Labriola JE, Lee TQ, Debski RE, et al. Stability and instability of the glenohumeral joint: The role of shoulder muscles. J Shoulder Elbow Surg 2005;14:32S-38S. [Crossref] [PubMed]
- Luime JJ, Koes B, Hendriksen I, et al. Prevalence and incidence of shoulder pain in the general population; a systematic review. Scand J Rheumatol 2004;33:73-81. [Crossref] [PubMed]
- Nordqvist A, Petersson C. Incidence and causes of shoulder girdle injuries in an urban population. J Shoulder Elbow Surg 1995;4:107-12. [Crossref] [PubMed]
- Simonet WT, Melton L, Cofield R, et al. Incidence of anterior shoulder dislocation in Olmstead County, Minnesota. Clin Orthop Relat Res 1984;186-91. [PubMed]
- Browe DP, Rainis CA, McMahon PJ, et al. Injury to the anteroinferior glenohumeral capsule during anterior dislocation. Clin Biomech (Bristol, Avon) 2013;28:140-5. [Crossref] [PubMed]
- Konrad GG, Jolly JT, Labriola JE, et al. Thoracohumeral muscle activity alters glenohumeral joint biomechanics during active abduction. J Orthop Res 2006;24:748-56. [Crossref] [PubMed]
- Culham E, Peat M. Functional anatomy of the shoulder complex. J Orthop Sports Phys Ther 1993;18:342-50. [Crossref] [PubMed]
- Borstad JD, Ludewig PM. The effect of long versus short pectoralis minor resting length on scapular kinematics in healthy individuals. J Orthop Sports Phys Ther 2005;35:227-38. [Crossref] [PubMed]
- Moore KL, Agur AM, Dalley AF. editors. Clinically Oriented Anatomy. 4th ed. Philadelphia: Lippincott Williams & Williams, 1999:685-731.
- Bernstein J. editor. Musculoskeletal Medicine. Rosemont, IL: American Academy of Orthopaedic Surgeons, 2003:73-7.
- Phadke V, Camargo PR, Ludewig PM. Scapular and rotator cuff muscle activity during arm elevation: A review of normal function and alterations with shoulder impingement. Rev Bras Fisioter 2009;13:1-9. [Crossref] [PubMed]
- Harrold F, Wigderowitz C. A three-dimensional analysis of humeral head retroversion. J Shoulder Elbow Surg 2012;21:612-7. [Crossref] [PubMed]
- Hettrich CM, Boraiah S, Dyke J, et al. Quantitative assessment of the vascularity of the proximal part of the humerus. J Bone Joint Surg 2010;92:943-8. [Crossref] [PubMed]
- Zumstein V, Kraljevic M, Conzen A, et al. Thickness distribution of the glenohumeral joint cartilage: a quantitative study using computed topography. Surg Radiol Anat 2014;36:327-31. [Crossref] [PubMed]
- Robertson DD, Yuan J, Bigliani L, et al. Three-dimensional analysis of the proximal part of the humerus: Relevance to arthroplasty. J Bone Joint Surg 2000;82-A:1594-602. [Crossref] [PubMed]
- Angibaud L, Zuckerman J, Flurin P, et al. Reconstructing proximal humeral fractures using the bicipital groove as a landmark. Clin Orthop Relat Res 2007;168-74. [PubMed]
- Itamura J, Dietrick T, Roidis S, et al. Analysis of the bicipital groove as a landmark for humeral head replacement. J Shoulder Elbow Surg 2002;11:322-6. [Crossref] [PubMed]
- Wafae N, Atencio L, Vitor L, et al. Morphometry of the human bicipital groove (sulcus intertubercularis). J Shoulder Elbow Surg 2010;19:65-8. [Crossref] [PubMed]
- Kwon YW, Powell K, Yum J, et al. Use of three-dimensional computed topography for the analysis of the glenoid anatomy. J Shoulder Elbow Surg 2005;14:85-90. [Crossref] [PubMed]
- Yamamoto A, Massimini D, DiStefano J, et al. Glenohumeral contact pressure with simulated anterior labral and osseous defects in cadaveric shoulders before and after soft tissue repair. Am J Sports Med 2014;42:1947-54. [Crossref] [PubMed]
- Dunham KS, Bencardino J, Rokito A. Anatomic variants and pitfalls of the labrum, glenoid cartilage, and glenohumeral ligaments. Magn Reson Imaging Clin N Am 2012;20:213-28. [Crossref] [PubMed]
- Rao A, Kim T, Chronopoulos E, et al. Anatomical variants in the anterosuperior aspect of the glenoid labrum: A statistical analysis of seventy-three cases. J Bone Joint Surg 2003;85-A:653-9. [Crossref] [PubMed]
- Burkart AC, Debski R. Anatomy and function of the glenohumeral ligaments in anterior shoulder instability. Clin Orthop Relat Res 2002;32-9. [Crossref] [PubMed]
- Warner JJ, Deng X, Warren R, et al. Static capsuloligamentous restraints to superior-inferior translation of the glenohumeral joint. Am J Sports Med 1992;20:675-85. [Crossref] [PubMed]
- Ticker JB, Flatow E, Pawluk R, et al. The inferior glenohumeral ligament:a correlative investigation. J Shoulder Elbow Surg 2006;15:665-74. [Crossref] [PubMed]
- Moore SM, Stehle J, Rainis E, et al. The current anatomical description of the inferior glenohumeral ligament does not correlate with its functional role in positions of external rotation. J Orthop Res 2008;26:1598-604. [Crossref] [PubMed]
- Rainis EJ, Maas S, Henninger H, et al. Material properties of the axillary pouch of the glenohumeral capsule: Is isotropic material symmetry appropriate? J Biomech Eng 2009;131:031007 [Crossref] [PubMed]
- Moore SM, Ellis B, Weiss J, et al. The glenohumeral capsule should be evaluated as a sheet of fibrous tissue: a validated finite element model. Ann Biomed Eng 2010;38:66-76. [Crossref] [PubMed]
- Harryman DT, Sidles J, Clark J, et al. Translation of the humeral head on the glenoid with passive glenohumeral motion. J Bone Joint Surg 1990;72:1334-43. [Crossref] [PubMed]
- Mochizuki T, Sugaya H, Uomizu M, et al. Humeral insertion of the supraspinatus and infraspinatus: New anatomical findings regarding the footprint of the rotator cuff. J Bone Joint Surg 2008;90:962-9. [Crossref] [PubMed]
- Ackland DC, Pandy M. Lines of action and stabilization potential of the shoulder musculature. J Anat 2009;215:184-97. [Crossref] [PubMed]
- Thompson WO, Debski R, Boardman N, et al. A biomechanical analysis of rotator cuff deficiency in a cadaveric model. Am J Sports Med 1996;24:286-92. [Crossref] [PubMed]
- Tuoheti Y, Itoi E, Minagawa H, et al. Attachment types of the long head of the biceps tendon to the glenoid labrum and their relationships with the glenohumeral ligaments. Arthroscopy 2005;21:1242-9. [Crossref] [PubMed]
- Bain GI, Itoi E, Di Giacomo G, et al. editors. Normal and Pathological anatomy of the shoulder. Springer-Verlag Berline Heidelberg, 2015:275-8.
- Escamilla RF, Yamashiro K, Paulos L, et al. Shoulder muscle activity and function in common shoulder rehabilitation exercises. Sports Med 2009;39:663-85. [Crossref] [PubMed]
- Pagnani MJ, Deng XH, Warren RF, et al. Role of the long head of the biceps brachii in glenohumeral stability: A biomechanical study in cadavera. J Shoulder Elbow Surg 1996;5:255-62. [Crossref] [PubMed]
Cite this article as: Gasbarro G, Bondow B, Debski R. Clinical anatomy and stabilizers of the glenohumeral joint. Ann Joint 2017;2:58.